precisionmoldedplastics
457 posts
Don't wanna be here? Send us removal request.
Text
ADDITIVES FOR PLASTIC INJECTION MOLDING
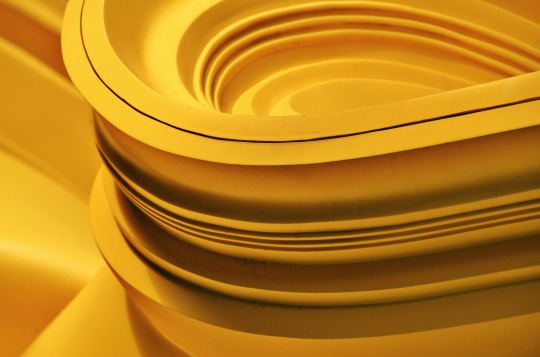
The plastics used for injection molding come in a variety of types, grades and chemical compositions. Each has its own unique properties and, thus, there are differences in their performance and in how they interact with the world. These resin characteristics are primarily why they are chosen as the base material for a particular part or component (cost being another common consideration). However, while a certain polymer may fit most of the requirements of a particular product, a need might exist for an additional quality the plastic does not contain. That’s where additives come in.
Additives for plastic injection molding are substances that are added to and bond with the base resin of a part in order to create a compound with certain modified or additional properties the basic polymer did not have by itself. Generally speaking, additives are used to enhance the appearance or performance of a part; to increase a material’s stability or flexibility; to facilitate or optimize production methods; or to extend the service life of a product. Further, additives have varying degrees of compatibility with different plastics (i.e., all additives will not mix well with or create the same qualities in all resins). A plastic additive can be in the form of a liquid, powder or pellet.
One note, by the way, is that plastic injection molding uses two types of materials - thermoplastics and thermosets. Thermoplastics can be melted and reshaped repeatedly, whereas thermosets are irreversibly hardened by curing from a soft solid or viscous liquid. Here, we will focus our attention on thermoplastics.
While there are myriad types of plastic additives, it can be helpful to visualize them in certain categories, although many can be placed into more than one classification, similar to movies fitting into multiple genres. With that being said, most additives fit into at least one of the following ten categories.
Light
Additives associated with light can be used to reflect, diffuse, filter, absorb or resist the harmful or aging effects of natural or other sources of light within various frequencies, wavelengths and amplitudes (e.g., infrared). In other words, these light stabilizers protect a part from photodegradation. Other materials also can be added to polymers to create a part that is laser markable.
Weather
Substances often are added to resins to guard against atmospheric conditions. These can include UV rays, radiation (i.e., electromagnetic waves), water, and extreme temperatures. Results can include a reduction in the alteration, fading or degradation of materials.
Temperature
Heat-related additives (e.g., ceramic fillers and mineral reinforced additives) include those that are fire retardant, flame resistant or that otherwise protect a part from high temperatures. Creating a higher melting temperature generally gives a material greater creep resistance (or a reduction in deformation under stress). Additionally, extreme cold can cause a plastic part to become brittle or crack. Accordingly, plasticizers, which increase the part’s flexibility and elasticity, or chemicals designed to lower a material’s freezing point (thereby adding to its stability) can be combined with a polymer. Thus, these types of additives can extend the operating temperature range of plastic materials. Also, some substances can increase a part’s thermal conductivity.
Processing
Some additives are included in a compound to assist with the injection molding process. These can include blowing agents, foaming agents, and mold release agents. Additionally, lubricants can be added to plastics to help with manufacturing or to increase the desired performance of the part.
Chemical
There are a variety of additives relating to resisting material degradation or contamination caused by other chemical agents, such as antioxidants and antimicrobials. In addition, some substances contribute to the chemical compatibility of parts used in the food and beverage, medical and other industries.
Moisture
Some additives are considered or used specifically in relation to water, whether in its liquid or gas (vapor) form. These include moisture resistant or repellent substances, which can provide anti-stain, non-leaching and non-wearing attributes.
Physical
Many plastic additives are used to modify the physical, structural or mechanical properties of a part. These can be designed to increase the strength, hardness, softness, durability or rigidity of a resin. Alternatively, there are those that enhance a resin’s elasticity, flexibility or impact resistance. Also, some additives can be utilized to adjust the mass or weight of a part either up or down.
Visual
As a variation on some other categories, certain additives simply affect the appearance or aesthetic characteristics of a plastic part. Probably the most common of these are colorants - in the form of dyes and pigments - which can create a polymer in virtually any hue. In addition to standard colors, there are optical brighteners, those that glow in the dark, or those that create variegated effects (e.g., marbling), shimmer effects (e.g., pearlescent, iridescent), or sparkle effects (e.g., metallic, reflective). There also are thermochromic colorants, where colors change due to an increase or decrease in temperature, and photochromic colors that change with exposure to light. Likewise, some additives can modify the clarity of a part.
Bioplastics
Another relatively modern category of additives are bioplastics, which include biobased and biodegradable resins. Additionally, an assortment of recycled materials, agricultural bioproducts, natural fibers and biocomposites are being added to traditional plastics in order to create a more eco-friendly end product. Bioresins themselves also can serve as the base material for a part.
Electrical
Certain plastics need an additive to make parts antistatic or, alternatively, to be electrically conductive. In addition, some components have security, traceability or identification requirements, where additives can provide X-ray detectability or radio-frequency identification (RFID), for example.
Conclusion
Plastic injection molding utilizes a wide range of polymers with various qualities and characteristics. However, many parts and components have specific requirements their base resins cannot precisely meet by themselves, despite otherwise being the best candidate for a product. When this occurs, substances collectively known as additives can be blended with the basic material to create a compound with modified or added properties. Accordingly, plastic additives can optimize an injection molded part’s performance or appearance, and they should be considered when designing and developing a new project.
–
Want more information?
Check out this supplementary infographic →
Questions?
Feel free to contact our support team. We’re here to help.
–
Ash Brown // VP, Business Development
#plasticinjectionmolding#injectionmolding#plastic#plastics#manufacturing#plastic additives market#plastic additives#additives
2 notes
·
View notes
Text
BIOPLASTICS & INJECTION MOLDING

As the interest in environmentally-friendly business and consumer practices increases, so does the desire for novel approaches and greater efforts towards addressing the issues surrounding plastic manufacturing, usage, waste, recycling and management. As part of that discussion, the term bioplastics is being used more frequently. What exactly does the expression mean, what are the issues and options associated with it, and how does it fit into a modern ecologically-sound commercial framework? As with most complex topics, the answers are somewhat complicated and are not unanimously agreed upon, although we attempt to review the basics in this article.
FACTORS
To begin with, there are two main factors associated with bioplastics. While often used somewhat synonymously, they actually are distinct concepts. The difference between the two ideas is fairly straightforward, as one deals with what can be referred to as the beginning-of-life of a material, with the other covering the end-of-life of a product.
Biobased
The first factor related to bioplastics is whether the material is biobased, which means the source is a biomass or that some or all the raw materials have a biological origin (i.e., plant or animal material). In other words, the source has a non-fossil origin. According to the U.S. Department of Agriculture (USDA), “Biobased content is how much ‘new’ or recent organic carbon is in an object or substance, compared to the amount of ‘old’ organic carbon it contains.” Put slightly differently, “the term biomass covers all materials of biological origin, apart from fossil materials and/or those incorporated into geological formations.” (Information Document 536 (EN) – 19.10, TUV Austria.) Thus, biobased products provide an alternative to conventional petroleum-derived products.
Basically, this refers to renewable resources (those that naturally can replenish themselves), as opposed to nonrenewable resources (those that are limited in supply and cannot be used sustainably), which include oil, natural gas, coal, and nuclear energy. Oil, natural gas and coal collectively are referred to as fossil fuels, which were formed from dead plants and animals over millions of years - hence the USDA’s reference to “new” vs. “old.”
Biodegradable
The second aspect of bioplastics is whether the substance is biodegradable. As defined by the International Union of Pure and Applied Chemistry (IUPAC), biodegradation is the “breakdown of a substance catalysed by enzymes [macromolecules] in vitro [in a laboratory] or in vivo [in a living body].” Generally speaking, a material is biodegradable “only if microbes [i.e., microorganisms or microscopic organisms] in the environment can break it down and use it as a food source.” Further, whether and to what extent a material is biodegradable depends on its molecular structure and not on its source. More specifically for our purposes here, a biodegradable plastic is “a plastic in which all the organic carbon can be converted into biomass, water, carbon dioxide, and/or methane via the action of naturally occurring microorganisms such as bacteria and fungi, in timeframes consistent with the ambient conditions of the disposal method.”
Additionally, although often used interchangeably, the terms biodegradable and compostable mean somewhat different things. While biodegradable means that a substance can decompose by bacteria or other living organisms, a compostable plastic is defined by the ASTM as “a plastic that undergoes degradation by biological processes during composting to yield carbon dioxide (CO2), water, inorganic compounds, and biomass at a rate consistent with other known compostable materials and that leaves no visible, distinguishable, or toxic residue.” (Emphasis added.) Thus, all compostable materials are biodegradable, but not all biodegradable materials are compostable. Additionally, most compostability definitions refer to industrial or commercial composting facilities, although some include home composting programs.
TYPES OF BIOPLASTICS
Using the two elements of biobased and biodegradable, there can be three different types of bioplastics.
1) Biobased Plastics. Resins that are biobased are derived in whole or in part from organic materials from plants or animals (i.e., a biomass). Not all biobased plastics are biodegradable.
2) Biodegradable Plastics. Plastics that are biodegradable can be chemically broken down by microorganisms in the environment within a limited period of time. Also, note that some biodegradable plastics have a non-biobased source, like petroleum, contrary to popular belief.
3) Biobased and Biodegradable Plastics. Some bioplastics are both biodegradable and are partially or wholly made from a biobased source.
BIOCOMPOSITES
Another type of material included within the category of bioplastics are compounds or composites containing a combination of substances.
A composite is a material that is produced from two or more constituent materials having different physical or chemical properties. Further, a biocomposite is formed by polymers derived from renewable (i.e., having a biological origin) and nonrenewable materials. In addition, composites usually include a combination of resin (a matrix or binding agent) and a fiber reinforcement, which can consist of either natural or synthetic fibers. Biocomposites generally contain a petroleum-based plastic and an organic filler, although some combine natural fibers with biobased or biodegradable resins. Further, natural fibers include wood fibers (recycled and non-recycled) and non-wood fibers, including straw, bast, leaf, seed, fruit, and grass.
RECYCLING PLASTICS
Another important topic included within the discussion on bioplastics is that of recycling. According to the CalRecycle Glossary of Waste Prevention Terms, the term recycling means “using waste as material to manufacture a new product. Recycling involves altering the physical form of an object or material and making a new object from the altered material.” Correspondingly, the ASTM defines recycled plastic as “those plastics composed of postconsumer material or recovered material only, or both, that may or may not have been subject to additional processing steps of the types used to make products such as recycled-regrind or reprocessed or reconstituted plastics.”
Generally speaking, there are three end-of-life options for recycling plastics - mechanical recycling, chemical recycling, and organic recycling. Mechanical recycling basically consists of melting plastic waste, which does not alter the molecular structure of the material. Chemical recycling modifies the plastic with the use of a chemical agent or process. Finally, organic recycling involves the disintegration of plastic materials in a municipal or industrial composting facility via aerobic (composting) or anaerobic (biomethanization) treatment. The output of these recycling efforts often consists of bioplastic granules that can be used to make plastic products (thus becoming a beginning-of-life option), thereby completing the circular recycling process or loop.
Additionally, according to the US Environmental Protection Agency (EPA), “The ability of biobased plastics to be recycled varies. Some forms of biobased plastic cannot be recycled together with petroleum-based plastics due to chemical structure incompatibility, while other biobased plastics may have compatible chemical structures that allow for recycling together with petroleum-based plastics.”
TESTING & CERTIFICATIONS
In the United States, there are certain standards, legal requirements and labeling guidelines relating to the different kinds of bioplastics.
Biobased Bioplastics
Because some bioplastics are not entirely made from a biomass, there are two approaches to analyzing their composition. First, you can look at the “biobased content, based on the amount of biomass in a product, taking account of the four key components: carbon, hydrogen, oxygen and nitrogen; The bio-based content is expressed as a percentage of the overall weight of the product in question.” Alternatively, “the biobased carbon content is focused on carbon and is generally expressed as a percentage of the carbon the product contains (organic carbon or total carbon).” (See ASTM D6866 and ISO 16620-1:2015.)
To determine the biobased content of a product and whether it can be certified as such, the USDA uses the requirements set forth in ASTM D6866. Additionally, as part of its BioPreferred program, the USDA maintains a voluntary labeling initiative where businesses may display the USDA Certified Biobased Product label on products that meet the USDA criteria for containing a verified amount of renewable biological ingredients (i.e., biobased content).
Compostable Bioplastics
In California and in some other states, it is illegal to use the term “biodegradable” in marketing claims related to plastic products, because the expression often is used to describe items that do not meet ASTM standards for compostability and, therefore, are contaminants for composters. In addition, for a label to state a product is “compostable” or “marine degradable,” that product must meet the applicable ASTM standard.
In the United States, for plastics to be considered compostable, they must be certified according to ASTM D6400 (or ASTM D6868 for biodegradable coatings). As stated by the standard, “The purpose of this specification is to establish standards for identifying products and materials that will compost satisfactorily in commercial and municipal composting facilities.” D6400 specifies three criteria for compostable plastics: (1) Disintegration; (2) Mineralization or Inherent Biodegradation; and (3) Safety considerations. It also creates labeling requirements for compostable materials.
Regarding labeling, the Biodegradable Products Institute (BPI) has created a compostable logo to place on products that meet the applicable ASTM specification. The BPI is the only third-party verification of ASTM standards for compostable products in North America. In addition, the Federal Trade Commission (FTC) has adopted the Guides for the Use of Environmental Marketing Claims or the Green Guides, which govern the marketing of environmentally friendly products and the use of certifications, seals and claims regarding the attributes of the associated products.
PURPORTED BENEFITS
Although a consensus does not appear to exist, there are many claims regarding the present and potential benefits of bioplastics. Some of the commonly discussed ones include:
• They save fossil resources by using biomass.
• Many are biodegradable.
• Bioplastic production consumes less fossil energy.
• Bioplastic production has fewer carbon dioxide emissions.
• Bioplastic production can help reduce dependence on imports and may create jobs and export opportunities.
• Use of bioplastics results in a reduction of our carbon footprint.
• Bioplastic usage reduces the global warming potential.
• Biobased products help us increase our use of renewable resources, while decreasing our use of non-renewable resources, such as petroleum.
• There is an alleged decrease in environmental toxicity.
• There is a potential reduction in litter and in the amount of trash sent to landfills.
• Bioplastics are cost-comparative, readily available, and perform as well or better than their petroleum-containing counterparts.
• The bioplastics industry is making a strong effort to use agricultural residues (cellulosics), other waste streams, and feedstocks that do not compete with food markets.
POSSIBLE ISSUES
In addition to the intended benefits of bioplastics, some people have expressed concerns regarding their impact on the environment or regarding their alleged advantage over other materials. Some of those issues include the following:
• Questions have been raised about the relative toxicity of bioplastics to conventional plastics.
• Some non-biobased plastics are biodegradable.
• Some biobased plastics are non-biodegradable.
• Bioplastic production consumes fossil energy and has carbon dioxide emissions.
• Bioplastics production results in pollutants, due to the fertilizers and pesticides used in growing the crops and the chemical processing needed to turn organic material into plastic.
• Bioplastics have some impact on food supply and availability.
• Most bioplastics can be broken down by microorganisms and become part of the natural world again in a short period of time, only if they are collected and composted in a carefully controlled, high-temperature industrial composting facility — and there aren’t many of those, especially in developing countries where the problem of plastic pollution is most severe.
• If bioplastics end up in landfills, as many do, without enough oxygen to break them down, they can last for centuries and release methane, a potent greenhouse gas.
• If bioplastics are thrown into the environment, they pose threats similar to other plastics.
• Many experts believe the solution to plastic waste mainly lies not in developing better bioplastics, but in overhauling the world’s economy to recycle far-greater quantities of plastic than currently are being reused.
To make these kinds of determinations, many analysts perform a Life Cycle Assessment (LCA), which is a data gathering and analysis tool that broadly assesses environmental benefits and burdens of a product. This approach is utilized by the EPA, for example. The procedures followed for an LCA often are those contained within the 14000 series of the International Organization for Standardization (ISO).
INJECTION MOLDING
Bioplastics can be used in many of the same ways as other plastics - for packaging, parts, components, disposables, durable goods, etc. Additionally, they can play a role in products associated with many different industries, such as agriculture, automotive, medical, food and beverage, and consumer goods.
When it comes to injection molding, bioplastics can have unique characteristics and perform in ways distinct from petroleum-based plastics. Thus, while the same general principles apply, to achieve quality, injection molded, bioplastic parts efficiently and consistently, some different approaches may need to be taken when building molds and certain modified processes utilized when manufacturing parts.
However, adequate experience working with these materials can result in producing parts historically thought to be achievable only by using traditional plastics. For example, Precision Molded Plastics manufactures bioplastic parts with durable living hinges (as shown in the image at the top of this article), which generally need to be made from polypropylene. Further, maintaining a good, working relationship with bioplastic manufacturers can be indispensable during the research and development phase of a new project using bioresins.
CONCLUSION
The awareness of environmental issues and desire to adopt ecologically-friendly business and lifestyle approaches is widespread and growing. As part of this trend, attention has been focused on plastic usage and waste management. Consequently, many in the public and private sectors have worked on developing sophisticated recycling systems. In addition, research into biobased and biodegradable materials and the manufacturing and utilization of bioresins have increased. While a complete consensus about the potential benefits of these materials may not exist, using bioplastics, coupled with greater recycling methodologies and adoption, may assist in achieving a cleaner world, a healthier population, and a better tomorrow.
Questions?
Feel free to contact our support team. We’re here to help.
--
Ash Brown // VP, Business Development
--
NOTICE
This article is intended to give a relatively academic and objective, although brief, summary of the topics and considerations related to bioplastics. It was not written or published to advocate for any specific viewpoint or for the use of any particular type of materials.
Virtually all products impact the environment. Accordingly, Precision Molded Plastics makes no express or implied claims regarding the biomass content, the biodegradability, or any environmental attributes or benefits of any of the products with which it is associated, or of bioplastics in general, or of any comparative advantage of bioplastics to other materials, and any such information must and should be obtained directly from the plastic resin manufacturers.
For more information, go to https://www.usda.gov, https://www.epa.gov, https://www.energy.gov, or https://www.calrecycle.ca.gov.
#bioplastic#bioplastics#bioresin#bioresins#biopolymer#biopolymers#plasticinjectionmolding#injectionmolding#recycling#sustainability#environment#ecology
1 note
·
View note
Text
THE BASICS OF CAD PART MODELING & FILE TYPES
Nowadays, computers are integrated into almost every aspect of our lives - from our cars, cameras and cell phones to our home appliances, business equipment, and the methods we use to obtain information and entertainment. The same is true for the design and fabrication of plastic parts, which used to entail a much greater level of manual input, creation and control.
However, despite the virtually ubiquitous use of computing in the engineering and manufacturing industries, some confusion and gaps in the public’s knowledge appear to exist, due in part to the rapid changes and innovations in the space. Accordingly, what follows should serve as a simple introduction to the basics of modern computer part modeling and file types.
SOME DEFINITIONS
Three-dimensional (3D) modeling is the process of developing a mathematical representation of any surface of an object in three dimensions using a collection of points in 3D space. Most 3D models are either (a) solid models that define the volume of the object they represent, or (b) shell models that represent only the surface of the object and not its volume, which commonly consist of polygonal meshes.
Computer-Aided Design (CAD) is the use of computers in the creation of a design, which includes 3D models and two-dimensional (2D) drawings (e.g., part prints). Computer-Aided Engineering (CAE) is the use of computer software (usually CAD) to aid in engineering analysis tasks, and Computer-Aided Manufacturing (CAM) is the use of software to control machines in the manufacturing of objects. CAD and CAM systems often are used to create programs to run Computer Numerical Control (CNC) machining equipment (like drills and lathes) and 3D printers. Additionally, a Coordinate Measuring Machine (CMM) is a device that measures the geometry of objects by sensing discrete points on the surface of the object with a mechanical or optical probe, which often is utilized for quality inspections of manufactured parts to verify dimensional conformity.
3D printing devices use digital computer models (usually mesh models) to form objects by printing material layer by layer. Another term for this production method is additive manufacturing.
Model-Based Definition (MBD) is the process of using an annotated 3D CAD model, which includes Product and Manufacturing Information (PMI) (i.e., any non-geometric data included within a 3D CAD file), as the definitive authority for downstream processes throughout the product life cycle. For example, in MBD, the information captured by the CAD software is fed into a CAM program, which creates computer code (usually G-code) to be executed by a CNC machine tool or other equipment. An important aspect of MBD is that data should be standardized, interoperable, and software (or CAx (Computer-Aided Technologies)) neutral. The digital data set may contain enough information to manufacture and inspect the object without the need for engineering drawings, although it can and often is used to generate them.
As mentioned above, G-code is the most widely used CNC programming language. G-code instructions are provided to a machine controller (i.e., an industrial computer) that tells the motors where to move, how fast to move, and what path to follow.
PMI can include Geometric Dimensions and Tolerances (GD&T), surface finish requirements, material specifications, and other data. Industry standards for defining PMI include ASME Y14.41 and ISO 1101:2012. Additionally, the National Institute of Standards and Technology (NIST) of the U.S. Department of Commerce has created a testing system to measure conformance of CAD software to ASME standards for PMI.
MODEL TYPES
Note that, while there are connections in this area with computer graphics software and modeling, our focus here is on engineering and manufacturing, particularly as they relate to the design and fabrication of plastic parts. That being said, much of the information contained herein may be applicable elsewhere.
Solid Models
Most CAE models originate from CAD systems, which typically use continuous surface and edge definitions based on a mathematical model called Non-Uniform Rational Basis Spline (NURBS). With NURBS, smooth surfaces and edges can be computed using a set of control points. NURBS is a type of curve modeling (which also includes geometric primitives and a few others), as opposed to polygonal or mesh modeling, as explained below.
Further, using a Boundary Representation (B-rep or BREP) method, a solid is represented as a collection of connected surface elements, which define the boundary between interior and exterior points. BREP is a type of geometry utilizing a set of NURBS surfaces seen as a solid. According to Wikipedia, “A boundary representation of a model comprises topological components (faces, edges and vertices) and the connections between them, along with geometric definitions for those components (surfaces, curves and points, respectively).” A vertex is a corner or point, an edge is a line segment between two faces connecting two vertices, and a face is a flat surface on a solid object. (ISO 10303-42 defines some data models for boundary representations.) Precise BREP solid models power most popular mechanical design tools, as they were the first technology to represent nominal geometry to within micron accuracy.
Another solid modeling technique is to use Constructive Solid Geometry (CSG), where 3D shapes are built by performing boolean operations (addition or subtraction) of simple shapes called primitives, such as cubes, cylinders, spheres, etc. In other words, CSG is the process of building solid objects from other solids. The three CSG operations are union, intersection and difference.
Shell or Mesh Models
In polygonal or mesh modeling, points (vertices) in 3D space are connected by line segments to form groups of polygons, or a mesh, to represent the surface or shape of an object. Mesh model files often are used by 3D printers, which deposit material layer by layer that becomes solidified into the object being produced.
Solid model files typically contain quite a bit of data and consequently require a considerable amount of computing power. Conversely, mesh model files generally are much smaller in size (unless the user is trying to compensate for the mesh’s poor representation of curved surfaces by increasing the file size). However, solid models can be converted into shell models that approximate certain features of the object, thereby reducing the file size and allowing computers to analyze and render them more quickly. Additionally, there are some conversion packages for turning mesh geometry into NURBS geometry, although they are somewhat limited and require a bit of work, as smooth, curved surfaces must be designed or modeled from scratch.
The process of transforming a mathematical representation of an object into a polygon representation is called tessellation, where objects are broken down (or approximated) from abstract representations to so-called meshes, which are nets of interconnected triangles or facets (i.e., flat surfaces on a geometric shape). Polygons are planar and only can approximate curved surfaces using many polygons. Additionally, all polygons can be made using triangles, so those are the most common shapes used. Therefore, a mathematical representation of a smooth, curved, continuous surface in three dimensions is approximated to a tessellated, triangulated, faceted, mesh, polygonal model of the surface.
Triangulated mesh surfaces describe surface geometry and shapes only. They are not precise, continuous boundary representations of the topological data of an object’s surfaces and curves (i.e., its faces, edges and vertices (or points)).
Wireframe Models
Wireframe models only include lines and curves that connect points (vertices), which thereby define the edges of an object. In other words, wireframes are just the outlines of a 3D object, and they do not include surfaces (faces), nor do they contain any mass properties. This is the least complex method for modeling 3D objects.
The Need for Precision
The advantage of having a mathematical definition of an object is that different levels of model detail can be produced without losing fidelity. When facets (polygons) alone are the geometric definition of a surface, producing different levels of detail causes aliasing problems (i.e., jagged or saw-toothed appearance of curved or diagonal lines), because facets cannot create a continuous, smooth surface. Instead, artifacts of the facets will manifest as sharp, abrupt changes, especially in regions of high curvature. No amount of mesh refinement can achieve smooth curvature.
In the disciplines of high precision engineering, manufacturing and measurement (e.g., plastic injection mold building and the molding and inspection of parts), the 3D models need to be smooth and precise at any scale. Therefore, formats using precise geometry are going to be the best fit for these tasks.
STANDARDIZATION, INTEROPERABILITY AND DATA EXCHANGE
In 1994, the International Standards Organization (ISO) established ISO 10303, which is a comprehensive standard for the representation and exchange of digital product and manufacturing information between computer systems. Its official title is “Automation systems and integration — Product data representation and exchange,” and it is known informally as “STEP” or the “STandard for the Exchange of Product model data.”
ISO 10303 includes numerous Application Protocols (AP), each of which define a data exchange standard for a certain family of products at a particular stage in its life cycle. Notably, AP203 defines the geometry, topology and configuration management data of solid models for mechanical parts and assemblies but does not include colors and layers. Additionally, AP214 includes everything AP203 does but adds colors, layers, geometric dimensioning and tolerance (GD&T), and design intent. (You may have noticed references to STEP AP203 and STEP AP214 in your CAD software, and this is why.) However, ISO has withdrawn both AP203 and AP214, which were integrated into AP242 to create a single standard for model-based 3D engineering. AP242 features more Model-Based Definition (MBD) support, such as PMI integrated with 3D models.
STEP files are used to represent part geometry and PMI that is used for data exchange and interoperability between CAD, CAM, CAE, CMM, and other computer-aided systems. STEP addresses product data from mechanical and electrical design, GD&T, analysis, and manufacturing, as well as additional information specific to various industries, such as automotive, aerospace, construction and others.
Native CAD file formats created by various modeling programs are proprietary and need to be translated and validated for use in other systems and for downstream processing. Conversely, neutral CAD file formats are interoperable between different computer software. The transfer and exchange of data is necessary so that, for example, one organization can develop a CAD model, while another performs analysis of the model, and a third is responsible for manufacturing the product.
In 2003, the American Society of Mechanical Engineers (ASME) published the ASME Y14.41-2003 Digital Product Definition Data Practices, which was later revised and is ASME Y14.41-2019. The standard provides for the use of many MBD aspects, such as GD&T display and other annotation behaviors within the solid model. ISO 16792 standardizes MBD within the ISO standards, sharing many similarities with the ASME standard.
COMMON FILE TYPES
There are many native or proprietary 3D modeling systems and file types, although most offer methods for exporting the native file into a standard neutral format. The most common neutral file types are as follows below.
Solid Model or NURBS File Types
In 1980, the Initial Graphics Exchange Specification (IGES) was established by the U.S. National Bureau of Standards (later renamed as NIST) as a vendor-neutral file format. The format still is used by certain industries, although interest has declined in recent years.
As previously mentioned, ISO 10303 instituted the STEP format for the representation and exchange of PMI. The STEP file format was intended to be a successor to IGES. (NIST actually provides a free STEP File Analyzer and Viewer program to use with these files.)
Other file types include the newer QIF (Quality Information Framework), described by ISO 23952:2020, and JT (Jupiter Tessellation), which is referenced in ISO 14306:2017 and mostly utilized by Siemens users. The Parasolid (X_T) format has been around for a while but is not a standard format, although it can be used by many modeling systems.
Mesh or Polygonal File Types
The most common universal mesh file format is STL (for stereolithography), which stores data based on triangulations of the surface of CAD models. Other common names include Standard Triangle Language or Standard Tessellation Language.
The Additive Manufacturing File (AMF) format was established by ISO/ASTM 52915. It is an open standard for describing objects for additive manufacturing processes, such as 3D printing, and is a successor to STL.
Another common polygonal file type is OBJ (object), which was first developed by Wavefront Technologies. It only represents the 3D geometry of an object.
CONCLUSION
The design, engineering, manufacturing and measurement of parts and components is performed almost entirely with the help of computer processing systems and software. The CAD modeling of objects includes mathematically precise solid models, polygonal or mesh models where curved surfaces are approximated with nets of interconnected facets, and wireframe models, which only define the edges of a 3D object.
Government agencies, standards organizations and trade associations have developed specifications for the representation and exchange of digital product and manufacturing data between computer systems with the intent of encouraging interoperability. Within this framework, standard neutral file formats for the different methods of part modeling have been established.
While the limited scope and context of this article preclude a thorough examination of these complex fields, having some basic knowledge of them should contribute towards the ability to ask the right questions of those performing the associated work, with understanding the answers given, and with determining any further research and analysis to conduct.
For more information, feel free to contact our support team. We're here to help.
Click here for a printable version of this article.
--
Ash Brown // VP, Business Development
#CAD#CAM#CAE#CNC#CMM#engineering#partdesign#3d model#3d modeling#STEP#MBD#manufacturing#injection molding#product development
1 note
·
View note
Photo
10 Stages in the Life of a Plastic Injection Molding Program
Thinking about launching a new product in 2021 that needs plastic injection molding? This infographic gives you an idea of what to expect for the lifespan of the program.
0 notes
Text
PART DECORATION FOR PLASTIC INJECTION MOLDING

Many plastic injection molded parts need to have some form of decoration applied to them after being manufactured. Possible reasons for decorating or marking parts include identification, tracking, branding, notices, warnings, instructions, as a product attribute, or otherwise. There are some standard methods of decorating plastic parts, and each has certain advantages and considerations, depending on the part application, the decorating purpose, and the resin being used.
PAD PRINTING
Historically, one of the more popular methods for decorating plastic parts has been with pad printing. There are three different types of pad printing machines: open inkwell, closed ink cup, and rotary gravure, which is used for printing 360 degrees on a part. Machines have a printing plate or a cliché, which commonly are made out of steel for durability. The artwork to be printed onto the parts is etched into the printing plate.
As the name suggests, the machines have transfer pads that come in a few different shapes and that are made out of silicone rubber with various hardness or shore levels. Pad life ranges from 10,000 to 100,000 cycles with an average of about 50,000.
The process consists of the pad picking up ink in the etched image on the cliché and placing it onto the part. Pad printing uses a type of solvent-based ink specifically designed for this purpose. This type of decoration also requires the fabrication of nesting fixtures to hold the parts in place during the printing process.
HEAT TRANSFER
A newer and usually more effective method of part decoration is thermal or heat transfer. With this process, the image is printed on a polyester film, which is coated with an adhesive so the image will stick to the part. Rolls of this film with the digitally printed images are placed on the printing machine’s reels, which index forward to print successive parts. Fixtures to hold parts are used here, as they are in pad printing. Additionally, a silicone decorating head or pad applies heat and pressure to print the transfer image onto the part.
Some advantages of heat transfer include the ability to create an image with four colors plus white in as high as 1200 dpi. In addition, there is no drying or curing time with decorating plastic parts, as there is with pad printing. Further, heat transfer decorations generally are tougher than pad printing and can be dishwasher safe.
HOT STAMP
Another common technique used to decorate plastic injection molded parts is hot stamping. A hot stamp machine transfers an image with pre-dried ink to a part with a die, again using heat and pressure and a fixture to hold the part, as with the previous methods.
One main advantage of hot stamping is the ability to apply a metallic foil or embossing to a plastic part. If you’re reading this article on a desktop computer, chances are the monitor in front of you has a logo that has been hot stamped on it. It also is a relatively clean process, compared to the wet ink used in pad printing.
LASER MARKING
Another method for decorating injection molded parts is by utilizing a laser marking system. This approach includes laser marking, laser engraving, and laser etching. Laser marking (or foaming) changes the color of the part where the text or graphic is, while the surface stays relatively unchanged. Alternatively, laser engraving removes some of the surface material, whereas laser etching melts the surface resin and creates a raised mark.
Of course, laser marking, engraving and etching all require a laser decorating machine. Additionally, fixtures to hold the parts usually are required, as with other decorating methods, although some fixtureless laser decorating systems have been developed. Some advantages of laser decorating include less wear and tear on the decorating equipment, it’s a precise and repeatable process, and it provides the ability to decorate some hard-to-reach part areas. Due to these benefits, industries like medical, automotive and aerospace often utilize the process.
IN-MOLD DECORATION
The method of in-mold decoration involves placing a pre-printed plastic film or appliqué in an injection mold before the part is molded, and the graphic then adheres to the part after it forms in the mold’s cavity. In-mold decoration requires a uniquely designed and built plastic injection mold and a carrier film feeder. The film can be manually placed into the mold between each cycle, but that greatly increases the manufacturing time and labor costs associated with the parts. However, even with a feeder, parts requiring in-mold decoration generally tend to be more expensive than other decorated parts, due to the specialized design, tooling and manufacturing required.
INKJET DECORATION
Inkjet printing on plastics is a relatively newer process, and it involves digitally printing a graphic onto a part. The system essentially is the same as inkjet printers for paper, with which most of us are familiar, although it uses a different type of ink. These machines typically use either conventional or LED lamps, and the process eliminates labels, films, printing plates, clichés or screens, as required with other decorating methods, although they do require a printhead and ink. Historically, this kind of decorating is somewhat slower and more costly than other forms, although advances are being made.
SCREEN PRINTING
Most people are familiar with screen printing in the context of t-shirts, and this also can be referred to as silk screening. Screen printing can be used for parts with flat or cylindrical surfaces but is not recommended for parts with complex curves and shapes. One advantage of screen printing is that it can print metallic graphics onto parts, which some other methods cannot do. Although providing the option of printing with multiple colors, a separate screen is needed for each separate hue, which increases cost and decorating time. The surface to be decorated needs to be prepared prior to printing, too.
PAINTING
When injection molding a part cannot achieve the desired texture, finish or color, painting the parts sometimes is utilized as a secondary operation. This approach can include spray painting and powder coating. As most injection molders do not perform painting as a finishing operation, the parts will need to go to a secondary provider, which often results in increased costs.
PLATING
When looking for a metal finish on an injection molded part, hard chrome plating and electroless nickel plating are two commonly used options. Plating offers a decorative finish, and it provides a certain level of corrosion protection. One item to note is that any surface imperfections on the part can affect the look of the plating, so consideration needs to be given there. Additionally, few molders also perform plating services, so those two suppliers will need to coordinate their work. There also are some design and material considerations that must be taken into account.
CONCLUSION
There are many different methods of decorating a plastic injection molded part, and each has its own unique advantages and disadvantages, depending on the requirements of the project. When developing a new product with components that may need to be decorated in some way, attention should be given to the part design, the polymer being used, the decorating purpose, the part application, the governing regulations, and the cost considerations. If we can answer any questions, don’t hesitate to reach out to us.
--
Ash Brown // VP, Business Development
1 note
·
View note
Text
ECONOMIC ORDER QUANTITY

Determining the best approach toward purchasing materials from your suppliers can be challenging. How often should you order, and how many parts should you order at a time? What’s too much, and what’s too little? You don’t want to have a warehouse full of unused items, but you don’t want to run out of product, either. Luckily, there’s a relatively straightforward method for calculating this.
The Economic Order Quantity (EOQ) is a formula designed to identify the optimal amount of goods to order that minimizes costs related to purchasing, delivery, receiving and storage of inventory while concurrently matching demand and, thus, maximizing profitability. This model relates to inventory management, production scheduling, and cash flow, and it helps to determine a company’s inventory reorder point. Additionally, it assumes the demand rate, ordering habits, holding costs, and lead times all remain constant. Generally speaking, the analysis is performed based on annual numbers.
Purchasing costs are those related to the actual placing and processing of an order for product. Outbound handling, shipping and receiving of orders have direct and ancillary expenses, too. Additionally, holding or carrying costs refers to the expense of storing inventory, which includes those related to shrink (stolen or missing goods), scrap (damaged goods), the actual storage space, utilities, labor, and insurance. These costs also include the time and resources spent and associated with performing the task.
The goal here is to determine your Goldilocks point for ordering parts. Order too many parts, and they become dollar bills sitting on warehouse shelves collecting dust, instead of providing the company some value elsewhere (i.e., opportunity costs). However, ordering too few parts can result in shortages, delays, unhappy customers, and lost profits. So, the desire is to order the right amount to meet demand while minimizing costs.
The EOQ is calculated by using the following formula:
EOQ = √2DS/H
The variables in the formula are as follows:
D = Demand in units (or estimated annual usage) S = Order cost (per purchase order) H = Holding or carrying costs (per unit, per year)
So, to use a fairly simple example, if the annual demand is 2,500 units, the cost per purchase order is $10, and the annual holding cost per unit is $5, the EOQ formula would be applied like this:
(2 x 2,500 x $10) / $5 50,000 / $5 10,000 Square root of 10,000 = 100 EOQ
Therefore, by ordering 100 units every time you place an order, you will minimize your costs while meeting demand, provided the assumptions mentioned above hold true. In addition, this allows us to compute the number of orders to be placed each year. Using the same example, that would be done like this:
Annual Demand / EOQ 2,500 / 100 25 orders per year
For quick computations, check out this online EOQ calculator.
Finally, there are some limitations with this approach in that some of the elements (e.g., customer demand) actually do fluctuate in the real world, potential quality issues are not included, and other variables, like quantity discounts, can affect the analysis. Nevertheless, the presumptions do not have to be exact, and allowances can be made for variations. Fundamentally, just having a basic understanding of the Economic Order Quantity model should contribute to more efficient materials purchasing and more effective inventory management, which results in greater overall profitability.
--
Ash Brown // VP, Business Development
#supply#supplychain#sourcing#procurement#purchasing#manufacturing#inventory#inventory management#injectionmolding#plastic injection molding#economic order quantity
0 notes
Text
INTERCHANGEABLE INSERTS

What do you do when you have a part to be injection molded, but it needs to be in a couple of slightly different sizes? Or, how about having different versions of a part where some features move locations or change just a bit? In other words, what are the tooling options for similar but differing parts? Of course, you can’t change the size or configuration of a steel mold... Or, can you?
Naturally, one approach to molding different, but not identical, parts is to build separate molds for each. Alternatively, a family mold with cavities and cores for each part could be built, too. However, one option is to build only one mold that has interchangeable inserts.
A plastic injection mold with interchangeable inserts has different, removable, internal cavities and cores that bolt into the mold base. This can be a cost-effective approach to tooling and molding for multiple parts with slightly different sizes and configurations.
One of the clear cost savings regarding tooling comes from the fact that only one mold base and set of internal components has to be purchased, although the materials for each cavity and core must be obtained (of course). Also, the mold builder doesn’t have to perform all the work to fabricate several different molds but only needs to create one plus the necessary inserts. So, the time and labor to build one mold with interchangeable inserts should be quite a bit less than it would be to make many complete molds.
On the manufacturing side, having one mold that produces a part with multiple variations by using different inserts can save time on production, because only one complete mold set-up is required. Obviously, the inserts need to be changed between production runs, but that generally is much quicker than taking a mold out of a machine and installing a completely new one, considering all that entails (e.g., placing the mold in the machine with a forklift or crane, hooking up water lines).
While interchangeable inserts may present some advantages to other methods toward molding similar parts, there are some caveats to keep in mind. First, not all parts with related features are good candidates for this approach, because their unique geometries might preclude it for some reason. Additionally, when utilizing interchangeable inserts, the different parts will be produced consecutively, whereas they would be made concurrently using a family mold, for instance. So, the manufacturing time will be longer, which might affect part prices. Also, if the demand is too high for the parts, this method might not be practical, although it may fit into a bridge tooling approach nicely, at the beginning of a program.
Further, if you are considering having a mold with interchangeable inserts built, make sure the inserts can be changed while the mold is in the molding machine, if at all possible. If the mold needs to be removed from the machine, taken to the tooling department for the insert change, and then brought back to the production floor and re-set up, that clearly requires additional, wasted labor and time, which eliminates much of the manufacturing efficiencies achieved otherwise.
In sum, for a part with several similar versions and relatively moderate production demand, building and using a plastic injection mold with interchangeable inserts sometimes provides certain efficiencies and economies. Accordingly, keep the option in mind in case the opportunity ever presents itself.
If you would like more information on this subject or on anything else related to plastic injection molding, visit our Resource Center or please don’t hesitate to contact us. We look forward to speaking with you.
--
Ash Brown // VP, Business Development
0 notes
Text
WHAT TO EXPECT REGARDING LEAD TIMES ASSOCIATED WITH PLASTIC INJECTION MOLDING

You’ve been given a new project to source that includes some plastic injection molded parts. If you don’t have a molding supplier (or if you’re not happy with the one you have, and this is the perfect opportunity to find a new one), it’s time to start doing a little research. While you certainly can find potential providers out there, just how long will it take for the bidding process? Once a contract is awarded, how long until the mold building can begin, and how long should you expect it to take until you get samples to inspect? After samples are approved, what kind of timeframe until the tooling is production ready? And, once the molds are complete, how long should it take to get parts?
Of course, all manufacturing programs are different, so each will have unique elements and will take varying amounts of time. Additionally, your company may have some specific sourcing requirements that vary from a standard approach, in which case the number of necessary steps, as well as the tasks within and timing of each, will be affected. Nevertheless, while the descriptions contained in this article are mostly estimates and generalizations, we can touch on the main points and walk through the process, so you have fairly accurate and reasonable expectations for each stage.
In addition, the research and development (R&D) phase of a new project can be very complex and literally take years. As such, we will begin with preparing for the bidding process and leave a discussion about R&D involving injection molding for another time.
RESEARCH & SOURCING
The search for a potential supplier can take months, possibly longer, depending on the complexity of the project. Finding the right molder with the necessary experience, qualifications, resources and offerings who is a good fit for the program and for your company generally takes some time. Unless you’re pressed for time (obviously not ideal), expect it to take a few months to make a somewhat lengthy list of potential molders and then a week or two to review the candidates internally and to whittle it down to your short list.
RFQ PREPARATION
To be as efficient as possible, it’s a good idea to start compiling the data and materials needed to create a detailed and thorough request for quote (RFQ) package while the sourcing research is being conducted. Performing both activities concurrently can save time, when and to the extent it is practical to do so.
Further, the investment required for tooling used in plastic injection molding can be significant. Thus, it is well worth the time and effort to review all relevant factors when preparing an RFQ and making sure it includes all the essential elements. Accordingly, just gathering the necessary items can take weeks, as will creating the production files and drawings, if they don’t exist, although we will assume they do for this discussion. Then, it can take days or even weeks (depending on the project’s complexity) to draft an organized request, including all applicable information, summarizing the project, etc.
BIDDING PROCESS
Although making plastic parts might seem fairly straightforward to some, modern mold building, scientific injection molding, and professional quality control procedures involve multiple disciplines and require a significant level of expertise to perform properly. As such, preparing a thorough and accurate proposal for building molds and/or manufacturing parts should involve reviewing and analyzing quite a bit of data and collaborating with the customer to customize the approaches taken in order to optimize the program. Therefore, it should take at least a week for a good supplier to bid on a job, but it can take a month or two depending on the complexity of the project.
TOOLING DESIGN & ENGINEERING
An important element of building an injection mold is performing a Design for Manufacturability (DFM) study on the parts to be manufactured. A DFM study analyzes the design of a part with the intent of optimizing the quality of the part and the efficiency of the manufacturing process. Performing the study with the proper software and then reviewing the study report with the customer generally takes about a week. If the DFM study identifies anything other than minor recommended design changes, the time needed to make major adjustments often takes weeks. Subsequently, another DFM study may be warranted, further design changes made, etc.
After the part design is finalized, most tool builders prepare a mold design based on the approved DFM report and review the mold design with the customer. This often takes a week or more.
MOLD BUILDING
Once the DFM study report and mold design has been approved, the building of the mold can begin. This includes purchasing materials and performing the actual CNC work, EDM, milling, assembly, etc. Of course, the time before work actually starts depends on the lead time for receiving materials and on the mold builder’s schedule. Generally speaking, fabricating a custom plastic injection mold will take a minimum of three months, although the time varies greatly depending on the complexity of the mold. Single cavity tooling without side action can take a little less time, while multiple cavity molds with slides or other mechanical elements can require a much greater period.
MOLD QUALIFICATION
Once the fabrication work is complete and the mold has been assembled, the builder will perform the mold qualification testing to confirm the dimensional accuracy of the part and to validate proper mold function. Once parts produced during this process meet the dimensional requirements, these “tooling samples” should be provided to the customer along with an inspection report. The mold qualification stage, as before, depends on the builder’s schedule, although it often takes about a week or two, assuming adequate planning.
If the samples are not approved, the mold needs to be disassembled and modification work is performed. Alternatively, the parts may be conforming but some issue exists, so a part design change might be needed. That could take a significant amount of time, depending on the work required to be performed on the mold, which might be quite a bit and possibly entail ordering new materials, even for seemingly minor design changes.
Subsequently, the mold is reassembled, retested and more samples are produced. The modification work and another round of testing generally takes at least a couple of weeks, although it depends on the severity of the changes needing to be made. Also, if more materials need to be ordered, that could delay things a bit further based on the material supplier’s lead time.
TOOLING COMPLETION & SHIPPING
As soon as the tooling samples are approved, the builder can perform any remaining work on the tool (e.g., polishing, mold labeling), prepare it for shipment (assuming the builder and the molder are different), and then ship the mold to the molder. Expect that finishing work and prep time to take around two to three weeks. The actual time it takes to ship the tool to the molding supplier depends on the distance traveled. A safe estimate for a shipment from overseas is about a month, plus outbound customs at the place of origin and inbound customs in the US often take about a week each.
PROCESS VALIDATION
Once the tool hits the molder’s dock, they generally perform an inspection, clean the mold (builders usually apply some type of anti-rust grease, when traveling over water), and prepare it for testing, all of which takes a week or so, depending on their current schedule. When the molder has the necessary materials, the mold will go into a molding machine for testing. This procedure is referred to as process validation, which establishes the optimal process parameters for a particular mold, so that it consistently produces parts that conform to the specifications. This can be a lengthy process to perform, so expect it to take at least a week.
The result of this step is the production of first article samples. Once the molder performs their quality inspections and the samples are conforming, they will provide them to you along with the inspection report. If the first articles are approved, the mold should be ready to go into production. However, if there are issues, the process may need to be manipulated, which could take a few days, or it could be something more serious, which might take longer to remedy.
ORDER PROCESSING
Once a mold is placed into production, it usually is stored at the molder’s facility for quick and easy access when orders for parts are submitted. When a purchase order is received, a molder needs to verify terms, pricing and its inventory of raw material, finished goods and other associated items (e.g., packaging) and put the order on its production schedule. The time a molder takes to process and fill orders can vary, and most suppliers have stated policies, (which is good to get in writing). Nevertheless, production should begin for new orders within four weeks, unless there are unique circumstances.
PRODUCTION
While a molder can state their policy for the time it will take to start manufacturing parts after their receipt of an order, the duration of each production run, obviously, depends on the size of the order and on the cycle time for the mold. For example, an order for 10,000 pieces with a cycle time of 20 seconds will be done quicker than an order for 100,000 pieces with a 30 second cycle.
Prior to production, the mold needs to be retrieved from storage and placed into the machine, the machine needs to be cleaned from the last production run, the new material needs to be put into the machine, and the process identified during the process validation phase must be established. (There are more steps involved, but we’re just mentioning the main ones.) During and after production, quality inspections take place. If any secondary operations (e.g., machining, decoration, assembly) are needed, those are performed at the appropriate times and inspections should be performed afterwards. Once everything is completed, parts will be packaged, placed on pallets, and shipped from the supplier. Due to the number of variables here, giving an estimate of time would be difficult.
MOLD TRANSFERS
If tooling already exists and is being moved to a new supplier, the timing analysis basically will start with the Process Validation stage, although the mold inspection performed might be a little more in depth. The actual time involved with transferring molds can be fairly short, especially with a little upfront planning. Additionally, offering to buy any existing material from the incumbent molder creates some good will with them, because they literally won’t be left holding the bag, if they had to purchase specialty material to make your parts and they have some left in inventory. This saves time in that they may not drag their feet as much to get your mold ready to ship, and it gives the new molder the exact material they need to make parts, which should save some time, too.
CONCLUSION
Mold building and plastic injection molding are complex undertakings. Accordingly, professional suppliers will require a significant amount of data to perform their responsibilities properly, and it often takes a sizable amount of time to analyze and understand the applicable information, to collaborate with the customer and all relevant third parties, to obtain the necessary materials, to manufacture the item in question, to test and inspect it, and then to ship it to its destination.
Furthermore, every molding and manufacturing project is unique and, as such, the total time they take will vary, as will the duration of each constituent step. Nonetheless, having at least a basic understanding of the approach, chronology and processes should establish reasonable expectations of the timeframe required for each, and we hope this article has aided in that way.
If you have any questions we can answer, please do not hesitate to contact us.
--
Ash Brown // VP, Business Development
#injectionmolding#plasticinjectionmolding#supply#supplychain#sourcing#procurement#purchasing#manufacturing#molding#moldmaking#tooling#engineering#leadtime#leadtimes
0 notes
Text
Time and Motion Studies

In addition to manufacturing, some plastic injection molders also provide assembly services. While there are many different types of product assemblies, there are some basic approaches towards determining what the process will entail. One of those approaches is referred to as a time and motion study.
For our purposes here, a time and motion study is a technique used to analyze both the duration each step in a manufacturing process will take coupled with the mechanical and manual labor involved to complete each task. By describing and studying an entire assembly procedure and each incremental step within it, a company is able to design, map, plan, budget, schedule, and standardize its processes effectively. Additionally, the more thorough the study is, the more accurately the quoting of the assembly services should be. Further, once an assembly process is established, periodic analyses can identify areas of improvement, which may include time efficiencies, the elimination of wasted motion, automating certain tasks, and an increase in the overall effectiveness of the system.
When conducting a time and motion study in the plastic injection molding context, the same general methodology is taken, while factoring in certain considerations unique to the industry. Regardless of the type of assembly required, one of the first steps in the analysis probably will include a determination of whether the assembly can be performed at some point during the production process and, if so, at what stage and location within the facilities the work can be conducted in the most efficient and effective manner.
Next, the supplier might work on the sequencing of the manufacturing and assembly processes. This calculation can be fairly simple, or it might require more complexity. For example, if the assembly a molder is performing only includes combining two different parts they are producing, the review might include which part to manufacture first; how to stage finished parts by the molding machine producing the second parts; what the method of affixing the two parts together entails (e.g., gluing); what equipment, tools and fixtures are required; what the estimated molding cycle time for the second part will be; any cooling or curing time needed for the either part; any necessary surface prep work; what movements will be involved in the actual bonding; any finishing work, labeling or cleaning required; what inspections are specified; and how to package the final assembly. And, that’s just a simple process! A more complex assembly procedure can involve multiple components, numerous steps, and the need for outside vendors.
Two obvious benefits to having your molder perform some or all of your product’s assembly is that it often is faster and cheaper than sending the molded parts, along with other items, to an outside assembly services vendor. Having parts and components combined by molding machine operators, who might be handling the parts as they are produced anyway, can eliminate unnecessary steps, such as additional packaging and shipping. This usually translates into lower costs and shorter lead times.
Product assembly can be a complex procedure involving multiple items, equipment and processes. As such, performing a thorough time and motion study at the beginning of a program launch can provide valuable perspective and expectations, in addition to discovering cost savings and lead time reductions. Accordingly, any accurate estimating of assembly services should employ this practice.
If you have parts being manufactured by a plastic injection molding supplier, be sure to inquire about any assembly services they might offer and how they arrive at their figures. You might be pleasantly surprised that the review of time and motion often translates into less time and money.
If you would like more information, please do not hesitate to contact us. We’re here to help.
--
Ash Brown // VP, Business Development
#injectionmolding#plasticinjectionmolding#timeandmotion#assembly#supply#supplychain#supply chain#sourcing#procurement#purchasing#manufacturing#engineering
0 notes
Text
Mold Qualification vs Process Validation

When a new plastic injection mold is built and initially assembled, it usually goes through two general stages of testing, inspections and evaluation. These phases serve somewhat different purposes, although they are related and both are necessary for the mold to be completed and put into production. Also, while certain projects and companies may require additional steps to be taken, the approach described here is common for most new tooling.
The first stage of testing new tooling customarily is referred to as mold qualification, which looks to see if part dimensions are to specification and if the part meets aesthetic and/or surface requirements. This examination is conducted by the mold builder, who subsequently provides some of the samples produced and the inspection reports containing their findings.
The parts produced during this process generally are called tooling samples. The tooling samples are inspected to confirm the dimensional accuracy of the parts (i.e., to make sure the measurements are within the allowable tolerances, especially with respect to any critical dimensions that have been identified) and to validate proper mold function. Samples produced during the mold qualification stage are not inspected for color, finish, etc., which will be done during the next phase of testing.
If any of the tooling samples’ dimensions are not to specification, the mold will go in for modifications, and another round of samples will be produced and inspected. Once everything meets the requirements, the mold can be completed, and the next stage of testing can be performed.
The second stage of inspecting new injection molds is called process validation. During the process validation phase, the molder establishes the optimal process parameters for a particular mold, so that it consistently produces parts that conform to the specifications. This can be a lengthy process, as arriving at an ideal molding process is a technical and specialized endeavor involving the interaction of multiple variables, including the injection speed, injection pressure, mold temperature, cavity pressure, cooling time, and ejection speed.
Process validation is conducted using the specific resin, colorant, additives and injection molding machine to be used during production. The parts produced at this point are called first article samples, which should be identical to the parts that will be manufactured once the mold goes into production and, thus, should be evaluated in every respect. To document the testing, a first article inspection report (or FAIR) will be prepared and submitted with the samples.
Similar to the mold qualification stage, if there are any issues, either the mold (occasionally) or the process (most likely) will need adjusting, and another round of samples will be created and inspected. Once conforming parts are produced, inspected and approved, the mold will be production ready.
Although this is only a short, simplified summary of the process for testing new plastic injection molds, it should give you a basic understanding of the steps and approach involved. If you would like more information, please do not hesitate to contact us. We’re here to help.
--
Ash Brown // VP, Business Development
#injectionmolding#plasticinjectionmolding#supply#supply chain#procurement#purchasing#sourcing#tooling#molding#engineering
0 notes
Text
TOP 20 THINGS TO LOOK FOR IN A PLASTIC INJECTION MOLDING SUPPLIER (PART 4 OF 4)

Part 4 of 4
This is the final installment in our four-part series on the qualities to look for in a plastic injection molding supplier. We hope you find it helpful in your research.
16. Business Knowledge
In addition to competency in manufacturing and in plastic injection molding, your supplier should know how to run a successful business. If they do, it should contribute toward their overall performance, which means higher quality parts, timely deliveries, helpful customer service, and competitive pricing. The perspective that comes from business and industry insight and understanding should result in a certain level of professionalism that makes them better and more of a valuable resource for you and for your company.
17. Guidance
Your injection molder should be an expert in their field and, as such, should provide you with the guidance necessary to make informed decisions regarding your parts and program. This can include optimizing part designs for moldability, manufacturability and performance; researching and recommending materials; and identifying potential cost saving initiatives. Additionally, as mentioned earlier, do they guide you through the problem-solving process when issues arise, and are they proactive in suggesting improvements before problems occur? Their knowledge, experience and expertise are a large part of what makes them a valuable resource to you and, consequently, should not be overlooked or undervalued.
18. Financial
Obviously, the process of finding the right injection molder will include a financial analysis. However, the bottom line often isn’t. There are many intangibles to factor in, and you need to decide how much you value each.
Additionally, make sure you are comparing apples to apples when looking at competing suppliers and proposals. For example, if tooling is being built, do the molds have the same number of cavities, the same warranties, the same expected lifespan, etc.? Is the molder providing you with different options for tooling and production and with quantity price breaks? Also, beware of any extra costs and fees, like mold qualification charges, process validation charges, mold setup fees, material and color change fees, outbound handling fees, etc. This is not to suggest those expenses are illegitimate, just make sure you factor them into your analysis.
19. Trust
As we all know, the foundation of any successful relationship includes trust. And, although this is related to other items on this list (e.g., dependability and the human element), we felt it was important enough to mention on its own. That’s because the value of truly believing your supplier will take care of you and your program cannot be overstated.
Do you trust the molder to look out for your best interests in a selfless manner, or do you think that, given the chance, they will take advantage of a situation to your detriment? There potentially will be opportunities for the supplier’s management and personnel to make decisions that will affect the quality and pricing of your parts. Likewise, knowing they will collaborate, communicate and cooperate with you means they truly are a business partner, which frees you up to focus on other suppliers of yours who aren’t.
20. Value
Not too long ago, I had an engineer tell me that the final decision on a project was not his and that, “Obviously, they [management] will go with whoever has the lowest price.” Unfortunately, this is an all-to-common and quite limited mindset, especially when considering the context.
When you are undertaking a project and engaging a company to provide you with custom manufacturing that is going to involve a significant financial investment and to require ongoing dependability over an extended period of time which could have a serious impact (positively or negatively) on your business’s sales, profitability and reputation, simply looking for and choosing the cheapest option is dangerously imprudent, incurs unnecessary risk, potentially hinders company growth, and generally invites problems.
Accordingly, while off-the-shelf parts may be just a commodity, the search for a supplier of custom injection molded parts should be focused on finding someone who provides the greatest comprehensive value. This must include analyzing all aspects of the molder as a supplier and as a business partner and evaluating how well they fit your wants and expectations, your company’s needs, and the unique requirements of the program.
–
Ash Brown // VP, Business Development
#plastic#injectionmolding#plasticinjectionmolding#supply#supplychain#sourcing#procurement#purchasing#manufacturing
0 notes
Text
TOP 20 THINGS TO LOOK FOR IN A PLASTIC INJECTION MOLDING SUPPLIER (PART 3 OF 4)

Part 3 of 4
Here are five more features of a potential molding partner to include in your research and analysis.
11. Standards & Certifications
Your company may have certain certifications its suppliers are required to have, so the molder obviously will need to have those. However, even if you don’t require anything specific, working with a supplier who maintains certifications or registrations with outside organizations and/or governmental agencies generally is a good idea, assuming you want to enter a relationship with a high-quality vendor.
Obtaining and maintaining certifications is no small feat so, presumably, the supplier earned whatever qualifications they have. Additionally, it is an indication the molder adheres to the applicable standards, because it undergoes audits conducted by objective third parties, in addition to internal reviews performed by its own staff. Quality certifications and standards, like those published by ISO, are the most common in manufacturing and can be general or specialized to a specific industry. Government agencies, most industries, and many trade associations have standards related to their respective fields, too.
12. Stability
If you are researching potential plastic suppliers, you are probably spending quite a bit of time and effort doing so. That’s because you want to make the best choice possible. Choosing an injection molding supplier is an important decision, because your company is entering into a potentially long-term relationship, not just a single transaction. Accordingly, one of the items to consider is the stability of the business.
Stability of a molder (or lack thereof) can manifest itself in many different areas. To begin with, how long have they been in business? It takes a lot for a company to remain in operation over a significant period of time. So, if they have been around for a while, they’re probably doing something right. Additionally, when you visit the plant (which you should), are the facilities clean, quiet and controlled? As Peter Drucker said, a dramatic factory is poorly managed. Also, what’s the general tone and demeanor of the molder’s staff? That question segues us nicely into the next item.
13. The Human Element
While, of course, this is a business-related subject and has to do with a business-to-business relationship, never underestimate the importance of people. Because, after all, most things basically boil down to people working together (i.e., human relationships). Assuming that’s true, what type of people own, manage and work for the molder? Are they friendly, helpful and accommodating? Are they authentic, empathic and ethical (as far as you can tell)?
These individuals will be the ones to receive and process your orders, to answer your calls and emails, to explain the causes of and solutions to any issues, to provide technical support, and to produce, package and ship your parts. They will make decisions that affect your program. Seeing as you’re entering into a long-term relationship with these people, do what you can to ensure they have the character and competence to handle your work properly.
14. Equipment & Resources
Although you may not need to get all the details regarding the molder’s equipment, obtaining some basic information can be insightful. Both quantity and quality are relevant here. For example, how many molding machines to they have, and how old are they? Also, what is their current workload capacity? What kind of quality inspection equipment to they use? Are they modern, digital vision instruments? If they have an in-house tooling department, what kind of equipment does it have? Additionally, is their equipment serviced and calibrated on a regular basis? Further, does the molder’s equipment look relatively new, clean, and well maintained?
15. Warranty/Guarantee
If the supplier is building tooling for your company, what kind of warranty or guarantee comes with it? Some mold builders don’t want to give any warranty, using a “we don’t know how you’re going to use it” type of argument. However, the same can be said for cars or any number of other products being sold in the world, so that mindset should raise some red flags. At the bare minimum, most goods sold in the US have implied warranties of merchantability and fitness for a particular purpose, unless expressly disclaimed. Molds should have certain warranties regarding materials (at minimum), craftsmanship (preferably), and completely for a certain number of cycles (ideally). Additionally, regarding the production and supply of parts, note the terms and conditions contained within the supply agreement regarding conformity, timeliness and any other relevant factors.
Check back next week for Part 4 of 4.
–
Ash Brown // VP, Business Development
#plastic#injectionmolding#plasticinjectionmolding#supply#supplychain#purchasing#procurement#sourcing#manufacturing
0 notes
Text
TOP 20 THINGS TO LOOK FOR IN A PLASTIC INJECTION MOLDING SUPPLIER (PART 2 OF 4)

Part 2 of 4
Searching for a new supplier takes time and effort. Trying to figure out how to measure and compare vendors can be challenging. To assist with this task, the first installment of this article presented five factors to consider in your analysis, and this part discusses five more.
6. Technical Support & Expertise
Plastic injection molding is quite a technical and specialized endeavor. Generally speaking, molders need to have a working knowledge of and experience with tooling fabrication and utilization; the chemistry and behavior of resin and additive types; utilizing machines with hundreds of tons of clamping pressure and inspection equipment to confirm tolerances of a thousandth of an inch; and efficient and effective production and quality procedures to manufacture and supply millions of conforming parts in a timely manner.
Accordingly, your supplier should be an expert that you can depend on to competently service the needs of your program and to advise you on virtually every aspect of your plastic injection molding program. At minimum, this includes part design, materials, tooling design and construction, engineering, processing, quality management, secondary operations, packaging, and logistics. In addition, suppliers should have personnel that are available and able to provide the necessary technical support you need. Further, your molder should attempt to be proactive and to identify and notify you of any issues before they occur and of any potential cost-saving measures that could be implemented.
7. Troubleshooting & Problem Solving
Even in ideal circumstances, stuff happens. When issues arise, does your molder have the knowledge and resources to identify the cause(s) and to implement solutions in a timely and effective manner? Do they consult with and explain things to you during the process? Do they have an in-house tooling department, or do they have to send the mold out for repairs? How quickly do they get the program back online? Problems are a part of doing business and, likewise, are an inevitable element of manufacturing. Accordingly, a plastic injection molder should be adept at troubleshooting and problem solving.
8. Dependability
We’re all busy. Trying to multitask. Hoping we don’t miss some deadline or forget to do something important. We have to-do lists and calendars and reminders and schedules and meetings and metrics and so on and so on. And, that’s just for work! As such, it’s crucial that you can rely on your molder to supply you with conforming parts on time on a regular basis, so you can focus your attention on other pressing matters.
Additionally, when those pesky issues arise (and they will), do you have the confidence in your molder to take care of it? The peace of mind knowing you have a dependable supplier can be incredibly important, as you may well know, especially if you have one that isn’t.
9. Continual Improvement
As the Red Queen said, “…it takes all the running you can do, to keep in the same place.” In other words, organisms (including companies) constantly must adapt, evolve and improve in order to survive and thrive against a constantly changing environment. As such, suppliers should have a practice of monitoring their performance, regularly reviewing its effectiveness, and implementing changes to keep up with the dynamic environment of business.
Also, is the molder certified to be operating in conformance with some industry standard, like ISO, which requires them to be continually improving? Do they communicate with their customers regarding their performance on an annual basis, at least, in the form of a customer satisfaction survey? Do they regularly add new equipment and service offerings?
10. Culture
Increasingly, company culture is becoming more important to employees, to customers, and to society in general. Corporate values and practices matter and, thus, should and do factor into buying decisions and brand loyalty.
Does the supplier appear to genuinely want to serve its clients? Do they have stated company values you agree with, and do they appear to follow them? How transparent are their operations? Do they have positive customer recommendations and testimonials? Has the company or its leadership been recognized as exemplary in any way? Use your intuition and try to gather as much objective evidence as possible, so that you can make the right decision.
Check back next week for Part 3 of 4.
–
Ash Brown // VP, Business Development
#plastic#injectionmolding#plasticinjectionmolding#supply#supplychain#procurement#purchasing#manufacturing
0 notes
Text
TOP 20 THINGS TO LOOK FOR IN A PLASTIC INJECTION MOLDING SUPPLIER (Part 1 of 4)

Introduction
Finding a custom plastic injection molding supplier can be a long and arduous task. You want someone who provides the most value and who is a good fit for you, for your company and for the program. Someone who checks all the boxes. But, what are those boxes, and how should you value each?
Ultimately, how you evaluate vendors and compare proposals depends on your unique needs and circumstances. That being said, this article is intended to offer some perspective to make the process a little easier, more thorough and, consequently, successful.
1. Service Oriented
Although supplying parts, viewed from a different perspective, a plastic injection molder provides the service of using your molds and their machines to convert plastic pellets into the shape specified by the product design. Therefore, as a service provider, it should be in a molder’s DNA to focus on serving their clients. Some elements of that would include accessibility, courtesy, patience, helpfulness, convenience, and professionalism.
2. Flexibility
As a corollary to service, your supplier should be willing and able to adapt to and accommodate the unique needs, requirements and specifications of your program, rather than having an overly rigid and uncompromising system and mindset. They should collaborate and cooperate with you in order to customize their services to meet your needs, as much as is reasonably practicable.
3. Communication
This can be viewed two ways: Procedurally and substantively. First, does the molder provide and maintain regular lines of communication? Nowadays, this means phone, email and text, at least, and can include instant messaging, enterprise communications (like Slack), and even on social media. Are they easy to get in touch with when you need them, or do you have to follow up to get a response? Do they respond to inquiries in a timely manner with valuable information?
Secondly, is your supplier effective at both verbal and written communication, being both thorough and concise in their explanations? Do they understand things from your perspective? Do they communicate with you in a way that makes it easy for you to understand the situation? Do they collaborate with you to resolve the issues?
4. Quality
Of course, as a manufacturer and supplier of parts and components, the quality of the fabricated product is of the utmost importance. Therefore, the molder should have a quality management system that is certified by an independent third party (e.g., ISO), which should give you confidence knowing they maintain a certain level of competency and performance. Do they have quality assurance and quality control procedures in place that they will customize to meet your requirements (reasonably speaking), and do they have knowledgeable quality personnel to carry out those processes? Do the molder’s employees undergo any type of training? Do they have a record of supplying conforming parts? How about positive customer testimonials and reviews?
5. Timeliness
Not only do you need good parts, but you need to get them on time. Consistently. Dependably. Do you receive complete, conforming orders in a timely manner, or are there delays and/or partial shipments? Additionally, what is the molder’s lead time for processing orders? Do they reply to inquiries in a timely fashion?
Check back next week for Part 2 of 4.
--
Ash Brown // VP, Business Development
#plastic#injectionmolding#plasticinjectionmolding#supply#supplychain#procurement#manufacturing#purchasing
0 notes
Text
Top 10 Reasons to Build Bridge Tooling

At the beginning of a plastic injection molding program, investing in bridge tooling (e.g., family molds) to bridge the gap between R&D/prototypes and multi-cavity production tools can be a good, cost-effective approach to take. Here are 10 reasons why.
1. It’s LESS EXPENSIVE to build fewer, low-volume molds, than it is to fabricate more, multi-cavity, high-volume tools
Fewer Molds 👉 Less Labor + Fewer Materials 👉 Lower Initial Investment
2. Bridge tools give you the ability to PRODUCE PARTS AT SCALE, which is cost prohibitive for methods like 3D printing
Generally speaking, injection molding is faster ⏩ and cheaper 💰 than additive manufacturing
3. It’s USUALLY QUICKER to get bridge tools built and validated, so you can get to market sooner
Fewer molds to build + Lower complexity tooling = Shorter build time
4. Selling your product sooner means having the ability to test the market and to start garnering MARKET SHARE and demand
Start selling 🏪 and marketing 📣 your product!
Find out what works 👍 and what doesn’t 👎!
5. Sales results in WORKING CAPITAL, which will help fund the fabrication of multiple cavity, high-volume molds later
Sales = 💲💲💲
6. Selling product will provide you with valuable MARKET FEEDBACK on functionality, issues, pricing, etc.
Your customers will tell you what they like, don’t like, and want! 🙂😐🙁
7. Actually molding parts will uncover areas of improvement in PART DESIGN, TOOLING AND MANUFACTURING
Your molder can identify 🔬 part and mold design changes 🛠️ to optimize part quality ⚙️ and the manufacturing process 🏭
8. Insights gleaned from the market and from suppliers can be used to REVISE AND IMPROVE your product
Designers 👩🎨, engineers 👨💻 and mold builders 👨🔧 can collaborate to improve 📈 the parts, processes and program
9. You can continue SELLING PRODUCT while designing, building and testing the multi-cavity, high-volume tools
Generate revenue 💵 and market the product 🗣️ while molds are being built 👩🏭 and validated 🏅
10. Once built and in production, using multi-cavity tools should result in INCREASED PROGRAM PROFITABILITY
Multiple cavity molds ➡️ Shorter manufacturing time ➡️ Lower part prices ➡️ Higher margins 😃
–
Ash Brown // VP, Business Development
0 notes
Text
CHOOSING THE RIGHT PLASTIC FOR INJECTION MOLDING

How do you identify the right resin for your plastic injection molded part? Beyond a certain plastic family, what grade, brand and characteristics do you need or want? On the other hand, if you already have selected a specific material, are there any alternative or equivalent resins available? If so, what are the differences and similarities, the pros and cons, the costs and benefits?
The base materials used for plastic injection molding generally are referred to as thermoplastic polymers. There are multiple broad families of plastic (e.g., ABS, Polypropylene, Nylon), and various grades with differing qualities within each family. Also, there are a myriad of brands of the same material family produced by different plastic manufacturers. Further, numerous additives exist that can be included with the base material in order to add or enhance a certain quality. Common examples include adding a UV additive to protect against harm caused by sunlight and using glass fiber to act as a reinforcing agent.
One of the first steps in choosing the right plastic for your part or component is to determine all of the applicable requirements. Legally speaking, any relevant statutory or regulatory requirements need to be identified. In the U.S., these can include federal and state laws, agency regulations, industry standards, and others.
Next, as a practical matter, what characteristics does the resin need to have? Some of the most frequently referenced properties of plastic used for injection molding include heat resistance, chemical resistance, impact resistance, weather resistance, UV resistance, moisture resistance, flame retardant, toughness, hardness, flexibility, stability, elasticity, strength, weight, mass density (or specific gravity), and clarity.
Once materials meeting the requirements are found, research can be done to identify any possible alternative or equivalent resins. On UL’s Prospector materials database (one of the most widely used, but subscription based), an alternative materials search will show numerous similar resins ranked on the correlation between the specified grade and the alternates regarding many different characteristics, including those mentioned previously and others relating to moldability. Moreover, if one digs into the details a little deeper, it may be seen that a possible substitute is even closer to the original material than reported, although Prospector did not have data for a certain material quality.
Armed with some potential candidates, the material suppliers (manufacturers and/or distributors) should be contacted regarding pricing, availability, lead times, and for their recommendations regarding any other possible substitutes you may not have uncovered. After receiving and analyzing all of that information, the next step would be to obtain some material from the supplier and then to use it to produce some sample parts. Different grades of material can be purchased and used to make samples in order to compare and contrast the properties of each resin. However, trying to use different material family types with the same mold can be problematic, due to the dissimilar rate of shrinkage between plastics, among other factors.
Generally speaking, the preferred way to determine the right plastic for any injection molded part, component or product is to have everyone associated with the program collaborate on the matter. At a minimum, supply chain personnel, engineers, mold builders, and injection molders all should be included. Each will bring his or her own unique perspective and, hopefully, add value to the discussion. Taking a joint, inclusive, multidisciplinary approach - where the different departments within a company and the customer and supplier all work together - should result in the identification and utilization of the right material for the job.
--
Ash Brown // VP, Business Development
0 notes
Text
The Relationship Between Mold Cavities and Part Price

When looking to get plastic injection molded parts or components made for a new project, buyers often make some kind of guesstimate about the number of cavities they want in the tooling. If the estimated annual usage (EAU) is fairly high, building a multi-cavity mold might make sense. However, there is a reasonably objective and fairly straightforward way to determine the number of cavities appropriate for a job, at a given stage of the program.
As a preliminary matter, it should be noted that, if a plastic injection mold is to have more than one cavity, it should have cavity balance. In other words, the number of cavities or flow groups (collections of cavities) on both sides of the sprue (the channel by which the molten plastic enters the mold) should be equal, and there should be a geometrically (or “naturally”) balanced runner system. This is necessary in order to achieve tight tolerances, a robust process, and fast cycle times. (The same general principle holds true for family molds, which make different parts, but we will limit our discussion here to those producing only one.)
The analysis for identifying the proper number of cavities in a mold begins with a determination of the estimated demand and the target part price. The demand essentially is a forecast of the number of parts needed per year and, consequently, the order amounts and frequency. For example, if the EAU is 120,000 parts per year, orders might be 10,000 every month; 20,000 every other month; 30,000 quarterly; etc. Target part price is determined by using whatever factors the buyer feels are relevant.
Once the demand is known, the amount of material needed to fill orders (i.e., the number of parts times the mass or weight of each part using the required resin) is calculated and communicated to the material suppliers, who respond with pricing, often including discounts at certain levels. Factoring in the cost of material at different order quantities, an evaluation can be done to see how many cavities are needed in order to hit the target part price. Possibly, one cavity will be sufficient. However, if not, two, four or more might be needed.
As you might guess, the main, underlying factor in the relationship between the number of cavities and the price of parts is time. If we assume a hypothetical production cycle time of thirty seconds, using a one-cavity mold, two parts would be manufactured every minute. Similarly, a two-cavity mold would produce four parts per minute, a four-cavity mold would produce eight, and so on. However, while this is roughly correct, it is not strictly accurate, as larger multi-cavity molds making more parts at a time require more plastic, which increases a few steps in the molding process, such as recovery time, injection time, and possibly cooling time (due to a larger runner).
Additionally, adding cavities to a mold usually increases its size, which results in it needing a larger molding machine in which to run. Further, making more parts at one time requires greater clamp pressure, also requiring a machine with greater tonnage. And, bigger machines are more expensive to run than smaller ones. Therefore, increasing the number of cavities does not necessarily reduce the part price to the same degree, although it does help.
Finally, building a bigger, multiple cavity mold requires more labor, time and materials than fabricating a smaller, single cavity mold. So, while the part price may decrease with a multi-cavity mold, the initial investment in tooling increases. Accordingly, at the beginning of a program, many companies choose to build smaller, less expensive molds and to pay a somewhat higher part price, and later they invest in multiple cavity tooling, once the program has obtained a certain market share and the company has the requisite capital to do so.
In conclusion, to determine the number of cavities needed in a plastic injection mold accurately and objectively, it is necessary to establish the estimated part demand and the target part price. Armed with those two items of information, an analysis can be conducted that will result in the required cavitation at given order quantities using the requisite resin.
How you approach the tooling needs for your program depends on these and on many other factors. If you would like some assistance in determining the proper path to take, please do not hesitate to contact us.
Ash Brown // VP, Business Development
0 notes