#Physical and logical qubits
Explore tagged Tumblr posts
Text
Amazon execs doubt Microsoft's quantum computing breakthrough
In This Story Final month, Microsoft (MSFT-1.51%) introduced that it created a brand new state of matter for its first quantum computing chip — a declare that Amazon (AMZN-1.15%) is reportedly not offered on. Trump freezes his 25% tariffs on Mexican imports for one month The identical day that Microsoft unveiled its Majorana 1 quantum computing chip, Amazon’s head of quantum applied sciences,…
#amazon#Amazon Web Services#Andy Jassy#Business#defense advanced research projects agency#Draft:Topoconductor#Finance#Google#IBM#Internet#Majorana#Majorana 1#Majorana 1 quantum computing chip#Matt Garman#Microsoft#Microsoft Azure Quantum#Oskar Painter#Physical and logical qubits#Quantum computing#Quartz#Satya Nadella#Simone Severini#Technology#Topological quantum computer
2 notes
·
View notes
Text
The Imaginative Quantum Cognition Theory
An amateur theory (mostly a draft) that combines the Quantum Cognition Hypothesis with Imagination - Originally written by yours truly, and rewritten in a more understandable way by ChatGPT
But First! What is Quantum Cognition?
Quantum cognition is the idea that human thought processes might follow the principles of quantum mechanics, rather than classical computation. It suggests that:
Superposition in Thought: The mind holds multiple possibilities at once before "collapsing" to a single decision, similar to how quantum particles exist in multiple states before being observed.
Quantum Probability in Decision-Making: Unlike classical logic, where choices follow strict cause-effect rules, quantum cognition allows for fluid, uncertain, and intuitive decision-making—much like how quantum systems behave probabilistically.
Entanglement of Ideas: Thoughts and memories could be connected in a way similar to quantum entanglement, where one idea influences another instantly, even if they seem unrelated.
This theory helps explain why human decision-making is often irrational, creative, and unpredictable, unlike the rigid logic of classical computers.
In simpler terms, it suggests that thoughts are actually a nebula of endless and unpredictable intel and the one we settle with is the thought our brains accept as the most probable/acceptable.
The Imaginative Quantum Cognition Theory aims to use the act of imagination as a proof for the "Quantum Cognition" hypothesis, and also try to figure out the unknown about imagination as a whole.
The Imaginative Quantum Cognition Theory is a speculative yet thought-provoking idea that suggests imagination and cognition function in a way similar to quantum computing. It proposes that thoughts exist in a superposition of possibilities, much like quantum bits (qubits), until they are observed and solidified into reality through creative expression. This hypothesis bridges the mysterious gap between neuroscience, quantum physics, and the nature of human creativity.
1. The Quantum Nature of Thought: Superposition and Imagination
In classical computing, a bit is either 0 or 1, meaning it holds a single state at a time. However, in quantum computing, qubits can exist in both 0 and 1 simultaneously due to a phenomenon called superposition. This allows quantum computers to process multiple possibilities at once, vastly increasing their efficiency.
Now, if we apply this to human cognition:
Imagination operates in a quantum-like manner. When we imagine, we hold multiple possibilities in our minds at once—an entire array of "what-ifs".
Thoughts exist in a probabilistic state until observed. When we consciously focus on a thought, we "collapse" it into a definite idea, much like how a quantum system collapses when observed.
Creative breakthroughs resemble quantum computation. The "Eureka!" moment of creativity might be the brain reaching the most probable and meaningful thought, much like a quantum computer finding the optimal solution.
Example: Think of a writer envisioning a story. At first, multiple possible plotlines coexist in their mind. As they refine the idea, weaker possibilities fade, and a single cohesive narrative emerges—just as quantum interference cancels out incorrect answers in quantum computation.
During spontaneous imagination, we (daydreamers) don't simply try out every single scenario and then decide which one is more entertaining or fits. We somehow calculate everything at the same time, our brains quickly guess the next action/scenery. If we make an error, we simply stop and go back. Notice how what determines the correct answer isn't eliminating all wrong ones but structuring the thought of "correctness" based on the current flow.
Engulfed in the flow, if we come across a correctness that feels more correct than the correctness we were previously looking for, we can even change our understanding of correctness and strive for that instead. For example, brainstorming. You think and think, if you reach something that is better than what you were previously looking for, you are very likely to abandon the previous "reality/goal" and adopt to the newest version.
2. The Fragility of Thought: Coherence and Decoherence in Imagination
In quantum mechanics, coherence is when quantum states remain stable, allowing complex calculations to take place. However, quantum states are fragile—any external disturbance causes decoherence, forcing the system into a definite state.
Similarly:
Imagination is fragile. Like a quantum state, a daydream or creative thought can vanish instantly if interrupted.
Focus maintains coherence. Sustained attention keeps the imagined idea in its fluid, multi-possibility state, allowing it to develop fully.
External distractions collapse ideas too soon. If a thought collapses prematurely due to distractions or mental fatigue, it may never reach its full form.
This might explain why deep daydreamers and highly creative individuals often need long periods of uninterrupted thought—their minds operate like quantum systems that need stability to fully process ideas.
Maladaptive Daydreaming may be the perfect example for this. They are fueled by music, creativity, and constant movement. Daydreaming takes extremely long and often ruins our perception of time and reality.
3. The Persistence of Ideas: Do Thoughts "Want" to Exist?
"I also noticed one more thing about imagination (and creativity) being quantum. Now, if we look at the way nature works, its primordial goal is to keep existing no matter what. Everything around us is built upon "reproduction" and "ignoring mortality" by constantly generating future generations, thus life never ends nor ceases from existence. Life enables this by wiring every single living being with the innate desire to reproduce at all costs."
"Can we connect the human desire to create art with quantum cognition's desire to keep existing? After all, the only way of making your thoughts turn into reality is by making them exist, either by recording (via writing, art, etc) or inventing them. Just like life, information doesn't want to disappear, I don't know why but it simply doesn't want to. For that, humans are equipped with the almost unbearable desire to make art, even though art is something that is nowhere near required to survive, yet it is a human need that can drive people crazy if it is lacking."
One of the most profound implications of this hypothesis is the idea that thoughts themselves may have an inherent drive to exist. In biology, life fights against entropy through reproduction. Could information, particularly imagined concepts, have a similar tendency?
Art as the DNA of Thought
Just as DNA ensures the survival of life, art ensures the survival of thought.
Ideas "fight" for existence by compelling their creators to materialize them into reality—whether through writing, painting, music, or invention.
Unexpressed ideas cause discomfort—much like unfulfilled genetic potential.
This could explain:
The deep urge to create despite art not being a survival necessity.
The haunting nature of abandoned ideas.
The obsession of artists who feel compelled to finish their work.
If this is true, then creativity isn’t just a luxury—it’s a biological imperative driven by the quantum nature of thought itself.
4. Maslow’s Hierarchy and the Quantum Drive for Creation
Maslow’s hierarchy of needs states that once basic survival needs are met, humans seek self-actualization—the need to express oneself fully and meaningfully. However, the Imaginative Quantum Cognition Hypothesis suggests that this drive might be more than psychological—it might be a fundamental law of thought itself.
The mind might be wired to turn abstract possibilities into reality.
Self-actualization could be the brain’s way of fulfilling the "quantum imperative"—the natural tendency of thoughts to collapse into reality.
The satisfaction of creativity could be the brain’s way of saying, "This was the most probable and meaningful outcome."
This aligns with the feeling of fulfillment creators experience when finishing a work—it's the moment when all probabilities collapse into a final, stable form.
5. Imagination as Quantum Computation: The Final Parallel
One final, profound question arises: If imagination works like quantum computation, are we the quantum observers of our own thoughts?
In quantum mechanics, the act of observation collapses a probability wave into reality.
In cognition, the act of focus collapses imagination into a concrete idea.
This could explain:
Why certain unfinished ideas feel disturbing—the quantum equation hasn’t fully collapsed yet.
Why some thoughts feel more real than others—perhaps they have stronger quantum coherence.
Why deep thinkers, writers, and artists struggle with intrusive ideas—their minds might be running high-powered quantum computations all the time.
If true, then the act of thinking itself is a form of quantum observation, shaping reality in a way that is yet to be fully understood.
Final Thoughts: What This Could Mean for Creativity and Reality
The Imaginative Quantum Cognition Hypothesis presents a radical yet intuitive way of understanding thought and imagination. If human cognition does, in some way, operate on quantum principles, it could mean:
Creativity is not random—it follows a structured quantum process.
Unexpressed thoughts are "fighting" to exist, much like life fights against extinction.
Reality itself may be shaped by how deeply and consistently we imagine it.
-2025
2 notes
·
View notes
Text
Atom Computing is Ushering in a New Era of Quantum Research

Atom Computing
Recently, quantum computers constructed from arrays of ultracold atoms have become a major contender in the race to produce machines powered by qubits that can surpass their classical counterparts in performance. Although the first completely functional quantum processors to be programmed via the cloud have been produced by alternative hardware architectures, further advancements indicate that atom-based platforms may be superior in terms of future scalability.
This scaling benefit results from the atomic qubits being exclusively cooled, trapped, and manipulated via photonic technology. Neutral-atom quantum computers can be primarily constructed using currently available optical components and systems that have already been optimised for accuracy and dependability, eschewing the need for intricate cryogenic systems or chip fabrication processes.
A physicist at Princeton University in the United States named Jeff Thompson and his team have been developing a quantum computer based on arrays of ytterbium atoms. “The traps are optical tweezers, the atoms are controlled with laser beams and the imaging is done with a camera,” Thompson explains. “The engineering that can be done with the optical system is the only thing limiting the scalability of the platform, and a lot of that work has already been done in the industry of optical components and megapixel devices.”
Enormous atomic arrays
Many attractive properties of neutral atoms make them suitable for quantum information encoding. Firstly, they are all the same, meaning that there is no need to tune or calibrate individual qubits because they are all flawless and devoid of any flaws that could be introduced during creation. Important quantum features like superposition and entanglement are preserved over sufficiently long periods to enable computation, and their quantum states and interactions are likewise well understood and characterised.
The pursuit of fault tolerance This important development made atomic qubits a competitive platform for digital quantum computing, spurring research teams and quantum companies to investigate and improve the efficiency of various atomic systems. Although rubidium remains a popular option, ytterbium is seen by certain groups to provide some important advantages for large-scale quantum computing. Thompson argues that because ytterbium has a nuclear spin of one half, the qubit can be encoded entirely in the nuclear spin.”They found that pure nuclear-spin qubits can maintain coherence times of many seconds without special procedures, even though all atom- or ion-based qubits havegood coherence by default.”
Examining rational qubits
In the meanwhile, Lukin’s Harvard group has perhaps made the closest approach to error-corrected quantum computing to yet, collaborating with a number of academic partners and the Boston-based startup QuEra Computing. Utilising so-called logical qubits, which distribute the quantum information among several physical qubits to reduce error effects, is a critical advancement.
One or two logical qubits have been produced in previous demonstrations using different hardware platforms, but Lukin and colleagues demonstrated by the end of 2023 that they could produce 48 logical qubits from 280 atomic qubits. They were able to move and operate each logical block as a single unit by using optical multiplexing to illuminate every rubidium atom inside a logical qubit with identical light beams. This hardware-efficient control technique stops mistakes in the physical qubits from growing into a logical defect since every atom in the logical block is treated separately.
The researchers additionally partitioned their design into three functional zones to enable more scalable processing of these logical qubits. The first is utilised to ensure that these stable quantum states are separated from processing mistakes in other sections of the hardware by manipulating and storing the logical qubits, coupled with a reservoir of physical qubits that may be called upon. Next, logical qubit pairs can be “shuttled” into the second entangling zone, where two-qubit gate operations are driven with fidelity exceeding 99.5% by a single excitation laser. Each gate operation’s result is measured in the final readout zone, which doesn’t interfere with the ongoing processing duties.
Future scalability Another noteworthy development is that QuEra has secured a multimillion-dollar contract at the UK’s National Quantum Computing Centre (NQCC) to construct a version of this logical processor. By March 2025, the national lab will have seven prototype quantum computers installed, including platforms that take advantage of superconducting qubits and trapped ions, as well as a neutral-atom system based on cesium from Infleqtion (previously ColdQuanta). The QuEra system will be one of these systems.
Replenishing the supply of atoms In order to create a path to larger-scale machines, the Atom Computing team has included additional optical technologies into its revised platform. Bloom states, “They could have just bought some really big lasers if They wanted to go from 100 to 1,000 qubits.” “However, they wanted to get the array on a path where they can keep expanding it to hundreds of thousands or even a million atoms without encountering problems with the laser power.”
Combining the atomic control offered by optical tweezers with the trapping capability of optical lattices which are primarily found in the most accurate atomic clocks in the world has been the solution for Atom Computing. By adding an optical buildup cavity to create constructive interference between multiple reflected laserThese optical lattices can improve their performance by creating a subwavelength grid of potential wells via laser beam interference.”With just a moderate amount of laser power, They can create a huge array of deep traps with these in-vacuum optics,” adds.”They could rise higher, but decided to show an arrangement that traps 1,225 ytterbium.”
Read more on Govindhtech.com
2 notes
·
View notes
Text
What I think I know about Quantum Mechanics in relation to Quantum Computing.
This will mainly be going off the top of my head because this is my current hyper fixation. I find it endlessly fascinating and seeing how you can break the veil of abstract and real and where you can in some instances literally see the effects. So what the shit does this have to do with computers. Classical computers use bits to do literally any function within any computer. It is a binary system 0 and 1 . On or Off. Once you get the hang of it you can write entire sentences in just binary, then convert that string into hexa-decimal binary to send a coded message that says "Poopy fart shit". Put that hexadecimal code into a .txt file and transfer it onto a floppy disk and hide in under the sys admins coffee mug, coaster style. Well Quantum Computers use a qubit. I am going to butcher whatever the fuck goes on with these so here is a definition from the internet. They are used to measure probability and calculate that within the infinity that lies between 0 and 1. "In quantum computing, a qubit (/ˈkjuːbɪt/) or quantum bit is a BASIC unit of quantum information—the quantum version of the classic binary bit physically realized with a two-state device. A qubit is a two-state (or two-level) quantum-mechanical system, one of the SIMPLIST quantum systems displaying the peculiarity of quantum mechanics."
Okay, my brain already hurts so fuck you I am done reading. Valid. You don't have to ingest or comprehend any of this long term. I just need to put it down somewhere. So for those along for the ride, lets a go! Okay to understand any of this we start with a classical bit. 0 or 1 . On or Off. When the logic of a program, for example Super Mario Bros. Within the games code there are variables or states a games code can be in. While the game is in various states like the Pause menu or the Start menu or the actual game. The NES and the game cartridge are running all of there pre-determined moves, music, and animation. There is one crucial understanding to all of this. While any computer is running it is WAITING for a binary input to interact with it. Computers WAIT for input both in classical and quantum. Each keystroke is sending one cycle 0 -> 1 then compounds and is translated by every component between the physical key switches and the html I am writing this on. This is all to state very abstractly that Classical Computing isn't simple, but it requires a circuit like understanding. If we are gonna talk quantum shit we gotta get some things cleared up. I am not an expert. Please prove me wrong if I say something incorrect. I am learning and trying to grasp these ideas. I appreciate you. Quantum Mechanics Time: No scary equations here, yet... However I want to clear up the stigma of the spooky math symbols. Everything has a definition and a rule set. What makes something quantum? A "something" is quantum if it can exist in what is called a superposition. That means being able to exist in two arbitrary positions in space at once, but only if it hasn't been measured. Once measured a quantum system or wave function collapses to a single point or in the case of a qubit state 0 or state 1. Woah I thought you said qubits are different I see a 0 AND a 1. Keen eye there sport! Keeping you on your toes. You see the magic happens behind the curtain. NOBODY SEE'S THE WIZARD you see? Before the wave function is collapsed/Qubit Measured the qubit did some funny business inside of the quantum computer. We gotta start from the very beginning so bear with me. Just like classical computers quantum computers wait for inputs to change states on the bit or qubit. So what causes the qubit to do the magic? Entanglement. When two qubits become entangled. No matter how far physically they are from each other. If you collapse the wave function of one the other immediately collapses as well. Not at the speed of light. INSTANTLY! This is done algebraically. You marry the two wave functions with math so if you change or measure one the change in immediate in the other. How does this work exactly? When you entangle 2 qubits you create a new wave function. This is due to the no-cloning rule of wave-functions. No wave functions can exist more than once. This is why we use complex numbers, you need the infinite obscurity as leverage. Once you can mathematically prove 1 measurement equals 2 queries. That means 2 is 4 and 3 is 9 and 4 is 16. Exponential. Next time on Quantum Computing. Bra-Ket Notation, and Matrix shenanigans.
10 notes
·
View notes
Text
Quantum Computing & Python: What Developers Need to Know
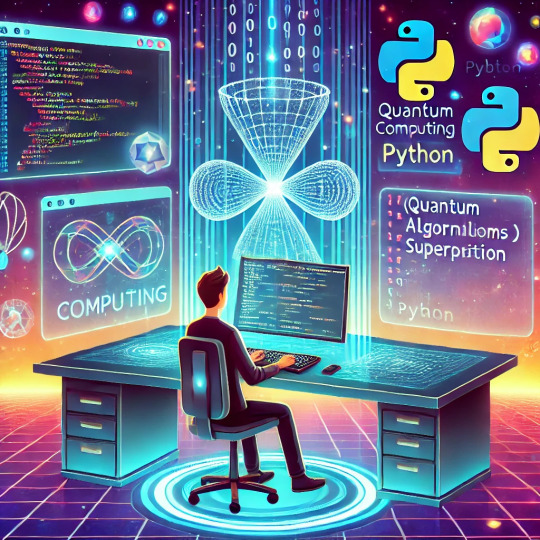
Quantum computing is one of the most exciting frontiers in technology, promising to revolutionize industries by solving problems that are currently impossible for classical computers. Python, being a versatile and widely used programming language, has become the go-to tool for quantum computing simulations and research. In this blog, we’ll explore the fundamentals of quantum computing, how Python fits into the ecosystem, and what developers should know to get started.
Understanding Quantum Computing Basics
Unlike classical computers that use bits (0s and 1s), quantum computers use qubits, which leverage the principles of superposition and entanglement to process information in fundamentally different ways. This allows quantum computers to perform calculations exponentially faster for specific tasks like cryptography, optimization, and complex simulations.
Superposition – A qubit can exist in multiple states (0 and 1) simultaneously, enabling parallel computations.
Entanglement – Qubits can be interconnected, meaning the state of one qubit can instantly influence another, even over vast distances.
Quantum Gates – Instead of traditional logic gates, quantum computing relies on quantum gates that manipulate qubits in unique ways.
Python’s Role in Quantum Computing
Python plays a crucial role in quantum computing, providing user-friendly libraries and frameworks for quantum programming. Here are some of the most popular quantum computing libraries in Python:
Qiskit (by IBM) – A powerful open-source framework for working with quantum computers and simulators.
Cirq (by Google) – Focuses on building, simulating, and running quantum circuits.
PennyLane (by Xanadu) – An advanced library for quantum machine learning.
QuTiP – Designed for quantum physics simulations.
Getting Started with Quantum Computing in Python
If you’re a Python developer looking to explore quantum computing, follow these steps:
Install Qiskit – pip install qiskit
Create a Simple Quantum Circuit
from qiskit import QuantumCircuit circuit = QuantumCircuit(2) circuit.h(0) # Apply Hadamard gate circuit.cx(0, 1) # Apply CNOT gate print(circuit)
Run on a Simulator or Real Quantum Computer – IBM provides free cloud access to quantum computers via IBM Quantum Experience.
Future of Quantum Computing
Quantum computing is still in its early stages, but its potential impact is vast. Fields like cryptography, AI, drug discovery, and climate modeling stand to benefit immensely. As quantum hardware advances, Python developers equipped with quantum programming skills will be in high demand.
Conclusion
Quantum computing is no longer just theoretical—it’s becoming an essential technology for the future. Python developers interested in innovation should start exploring quantum programming today. With tools like Qiskit and Cirq, diving into quantum computing has never been easier. Stay ahead of the curve and be part of the quantum revolution!
Python Training In Chennai
Python Course In Chennai
0 notes
Text
The Potential of Quantum Processing Units(QPUs): The Quantum Revolution

Quantum Processing Units (QPUs) are the key concept of a brand new way of computing. The principle of a quantum computer is actually, first of all, a qubit or a quantum bit. QPUs are using qubits which are the smallest subatomic particles that can be used to represent and process huge amounts of information ¹. Unlike the classical computers, QPUs use the principles of quantum mechanics to solve complex tasks which are otherwise intractable for the fastest supercomputers.
How QPUs Work
QPUs exploit qubits through quantum logic gates following the laws of the basic principles of quantum physics and making slight adjustments ¹. Thereby, QPUs decode information in a period of time close to what would take a classical computer years to accomplish. The use of quantum algorithms gives QPUs an advantage to run code in a different manner which makes them uniquely suited for complex tasks such as large number factoring and molecular simulation.
Key Principles of Quantum Computing
Superposition: Qubits can exist in many states at the same time.
Entanglement: Qubits become linked and can then transfer information instantly.
Decoherence: Qubits are reduced to a state where the measurement can be done, which is a non-quantum state.
Interference: Qubits are in a state where they can enhance or cancel.
Advantages of QPUs
Parallel Processing: QPUs conduct multiple calculations in parallel.
Exponential Scaling: The number of qubits can grow exponentially with the number of qubits involved.
Probabilistic Computing: QPs detect probable outcomes among several alternatives thus reducing the overall computational time.
Real-World Applications
Cryptography: QPUs have enough power to crack some encryption protocols.
Optimization: QPUs show a remarkable result in resolving complicated optimization problems.
Simulation: QPUs make a correct reflection of complicated systems.
Leave aside the future, the QPUs have very high potential, but they are still in their infancy. As research continues, we may look forward to QPUs being a tool for various areas like medicine, finance, and materials science ². Computing, as we know it can be a promising adventure, and by means of QPUs, we can be part of this.
#QuantumProcessingUnits#QPUs#QuantumComputing#QuantumTechnology#QuantumCollaborations#QuantumSecurity#QuantumPrivacy#QuantumEthics#Innovation#TechAdvancements
0 notes
Text
The future of quantum computing: Predictions for 2024

The field of quantum computing is expected to move from physical qubits to error-corrected logic qubits and increased global collaboration in quantum research in 2024, which will be a big year for the technology. Atom Computing's announcement of a 1,225-qubit quantum computer, almost three times larger than IBM's Osprey, which was the previous favourite, highlights that we are on the cusp of a new era in computing. The leap reinforces the potential for quantum computing to revolutionise industries such as pharmaceuticals and cybersecurity, enabling calculations of unimaginable complexity. However, it is increasingly believed that quantum computing will be driven by the quality of qubits rather than their quantity.
Quality of qubits over quantity
John Prisco believes that in 2024, the quantum computer market will see a shift away from an obsession with the concepts of quantum advantage and number of qubits. Instead, the industry will shift to fixing bugs and solving practical problems. "Quantum computing giants are expected to refocus their efforts on logical qubit counting, favouring qubit quality over quantity, which has dominated the debate so far. Unfortunately, while 2024 may not bring a decisive consolidation of quantum computer design modalities, this uncertainty calls into question the overall development and direction of the industry.
The quantum computer market will soon see one of the current modalities eliminated, meaning that 2024 will not bring a focus in development - a bad sign at a time when the industry is trying to move from high-profile feats to tangible impact on the real world. The transition from simply playing with numbers to persistently fixing bugs and building functional applications shows the maturity of the industry. This transition, while fraught with uncertainty, represents a critical juncture. As the industry struggles to translate theoretical possibilities into real-world applications, there is another looming challenge: the data security implications of quantum computing, particularly how hackers might use this nascent technology to store encrypted data today in order to decrypt it tomorrow.

Hackers will store data for later decryption
Fred Rivaine, CTO of Dashlane, makes a timely warning that quantum technology is evolving very quickly and the need for quantum-resistant encryption methods is no longer optional. The looming threat of a quantum computer breaking existing encryption standards, such as RSA or ECC encryption algorithms, highlights that organisations of any size and in any industry need to assess where they may be at risk and move to post-quantum cryptography today.
With the rise of quantum computing and artificial intelligence-driven attacks, a new generation of cyber threats will emerge. Cybersecurity teams will be challenged to defend not only against malware and traditional hacks, but also against quantum attacks focused on "save now - decrypt later," adversary machine learning tactics and AI-driven social engineering schemes. This will require cybersecurity professionals to have a broader skill set and better defence tools and platforms.
Misconceptions about quantum computing
There are many misconceptions about quantum computing. Tony Attlee, President and COO of Quantinuum, is quick to point out that quantum computers will not be around in ten or fifteen years. Already we have quantum computers that can do things that classical computers can't do." Another misconception is that quantum computers will only do one thing. Attlee explained that while the development of quantum computing has been primarily focused on business applications, the technology has unwittingly become an accelerator for solving complex scientific problems.
For example, problems that are often the domain of organisations such as CERN and projects such as the Large Hadron Collider can now be solved in ways previously thought impossible. Human history teaches us that if you give amazing tools to brilliant people, they will find what to do with them. We are seeing this in real time. In essence, quantum computing has become a transformative tool that can make theoretical concepts tangible, offering a new lens through which we can understand the universe."
Which industries will be affected by quantum computing?
Financial services, large banks and trading companies are using quantum capabilities to optimise their portfolios and improve fraud detection. The pharmaceutical sector relies on quantum computing for efficient drug discovery. At the same time, sustainability-focused companies are exploring new material sciences, as evidenced by BMW and Airbus' work on hydrogen fuel cells.
Quantum computing also promises to revolutionise regulated industries, from healthcare to aviation, by bringing much-needed transparency and traceability to AI algorithms, mitigating the black-box problem of AI decision-making. However, the risks are as great as the benefits. One is data encryption, as algorithms like Shor's algorithm have shown that quantum computing can break existing encryption methods. This raises not only technical but also ethical concerns about how to utilise the technology for the benefit of society while ensuring security.

What is the future of quantum computing?
Quantum computing is not a futuristic concept, but a current reality with key milestones on the near horizon. One of the near-term milestones is reaching a level where quantum machines will routinely outperform even the world's most advanced supercomputers in simulation tasks, bringing us into an era where classical computing will become incapable of emulating quantum capabilities.
We will begin to see it embedded in places that affect our daily lives. We may never touch a quantum computer ourselves, but it could be an app you're working on in your mobile phone that goes to the other side of the world and does something using a quantum computer. Like the early stages of the Internet and classical computing, quantum computing will start with niche applications, but it will soon become ubiquitous, quietly affecting the tasks we perform on our everyday devices such as mobile phones.
Challenges for the future of quantum computing
As quantum capabilities grow, we face an urgent need to invest in error correction mechanisms and focus on the quality of qubits rather than simple numerical superiority. Ignoring these subtleties could lead to a dangerous future in which the power of quantum computing is under- or mis-utilised. The path ahead therefore requires a calculated, ethical and interdisciplinary approach in which technological prowess and normative foresight converge.
Conclusion
As we approach 2024, quantum computing could quickly lead to a tipping point that will affect us all. Imagine faster drug discoveries or crack-proof passwords. But there's another side of the coin: Today's online security systems can be easily hacked, and the technology is still raw, often expensive and difficult to use. As we move from the old world of computing into the exciting but unpredictable quantum era, business and government leaders must take a big leap forward and prepare for potential pitfalls. In short, quantum computing opens up a world of extraordinary possibilities, but it is a tool that we must learn to handle with care.
0 notes
Photo
Advancing science: Microsoft and Quantinuum demonstrate the most reliable logical qubits on record with an error rate 800x better than physical qubits
0 notes
Text
The Quantum Computing Revolution: A Closer Look
Quantum computing, which harnesses the laws of quantum physics to process information exponentially faster than conventional transistor-based computers, is one of the most promising technologies of our time. It has the potential to revolutionize industries as diverse as agriculture, energy and financial markets. Yet as much as we know about quantum computing’s potential, it is also difficult to understand what the technology really means for businesses and consumers.
Developed in the 1990s, quantum computers are built on a fundamentally different basis than classical ones. Instead of relying on binary computer logic, which defines bits as either a zero or a one, quantum computers use the principles of quantum physics to create qubits, which can be both a zero and a one at the same time. As the qubits perform calculations, they can generate multiple outcomes simultaneously—a concept called superposition, which allows quantum computers to handle many more data points than classical computers, which must perform each calculation individually.

Scientists around the world are experimenting with developing hardware and algorithms to power these quantum machines, which have a unique capacity to process massive amounts of data in very little time. While the emergence of quantum computers has sparked excitement from technology website investors and entrepreneurs, it’s important to recognize that it’s still early days for this new technology. The development of quantum computers has been slow, and there are still challenges to be overcome if the full potential of this technology is to be realized.
A recent study, for example, revealed that quantum computing may not be as powerful as it originally seemed. Researchers found that the performance of quantum computers is influenced by factors such as temperature, vibrations and external electromagnetic fields. In addition, the system’s qubits must remain at a very low state of energy to keep them from losing their quantum properties, which can occur even when they are not performing computations.
As a result, the performance of quantum computers may only increase by about an order of magnitude over the next few years, at best. The research suggests that quantum computers will be able to tackle a limited number of problems, such as finding more efficient routes for shipping products to 100 cities or screening large numbers of small molecules for drug targets, but they will not reach the level needed to solve complex business and scientific questions.
Some experts, such techogle as Matthias Troyer, global head of group digital innovation at pharma giant Merck KGaA, Darmstadt, Germany, argue that the development of fault-tolerant quantum systems will be essential for businesses to take advantage of this new technology. “The reason we’re airing our skepticism about the impact of quantum computers is not to diminish their importance, but to ensure that researchers are focused on the areas where they have the most opportunity for impact,” he says.
1 note
·
View note
Text
qLDPC Library:Quantum Error-Correcting Code Research

Library qLDPC
Infleqtion researchers and JPMorgan Chase introduced a new open-source research software library today to speed up quantum application efficiency efforts. The Economist Commercialising Quantum conference in London on May 13–14 will provide more details on the news.
In conjunction with JPMorgan Chase, Infleqtion researchers created the open-source qLDPC library. Available on GitHub.
The main reason for this package was to help build and analyse quantum low density parity check (qLDPC) codes. However, the library tools also work for general error-correcting stabiliser and subsystem codes.
Reducing the number of physical qubits needed for quantum error correcting is one of the qLDPC library's biggest benefits. This library reduces fault-tolerant quantum computing hardware requirements by 10–100x. A single logical, error-corrected qubit used to need 1,500 physical qubits to work reliably. This new library may lower the requirement to 15–150 physical qubits per logical qubit, depending on implementation. This breakthrough fixes a scaling quantum system bottleneck.
The qLDPC library tools suit Infleqtion's neutral atom-based quantum computing technology. Infleqtion's hardware enables for extremely customisable qubit layouts, enabling the library's more efficient error-correcting codes.
The open-source library qLDPC is meant for collaboration. Developers, academics, and hardware partners can directly interact with the codebase to find new error correction and quantum workload optimisation approaches across platforms.
Important qLDPC library features include:
ClassicalCode: A class for classical linear error-correcting codes over finite fields, featuring pre-defined families and GAP/GUAVA package communication for more codes.
A class for creating stabiliser and subsystem Galois-qudit codes. Get_logical_ops, concatenate, and get_distance perform nontrivial logical Pauli operator construction, code concatenation, and code distance computation, respectively.
CSSCode: QuditCode subclass for building quantum CSS codes from two suitable ClassicalCodes. It uses research paper approaches to estimate code distance upper bounds in get_distance_bound.
Special quantum code constructs and family classes:
Two-block quantum codes.
BBCode, bivariate bicycle codes, including toric layout identification (like long-distance checks) and neutral atom qubit layouts that minimise communication distance. Several arXiv papers mention these constructs.
Product hypergraph codes.
Product codes for subsystem hypergraphs.
Subsystem hypergraph product codes simplex.
Lifted product codes.
Quantum Tanner codes.
decoders.py: BP-OSD, BP-LSD, belief-field, minimum-weight perfect matching, and additional error decoding modules.An interface for custom decoders is included.
Abstract algebra (groups, algebras, representations) module in Python. It communicates with GAP and GroupNames.org and uses SymPy pre-defined groups.
objects.py: A package for building quantum code auxiliary objects like Cayley and chain complexes.
qldpc.circuits.get_transversal_ops: A qubit code subroutine that constructs all SWAP-transversal logical Clifford gates in one code block, but it has exponential complexity and is more suitable for small-to-moderate codes.
Package requires Python >= 3.10 and can be installed via PyPI or source. C compilers for Windows and cvxpy for macOS may be required.
The project wants detailed documentation, however the current material is outdated. For help using the library's classes and methods, consult the source code, comments, examples directory, and test files.
#qLDPClibrary#quantumlowdensityparitycheck#qLDPC#quantumcode#QTCode#technology#technews#news#technologynews#technologytrends#govindhtech
0 notes
Quote
a new means of constructing a photonic quantum computer. Rather than using a single photon, the team employed a laser-generated light pulse that can consist of several photons. The research is published in the journal Science. "Our laser pulse was converted to a quantum optical state that gives us an inherent capacity to correct errors," stated Professor Peter van Loock of Mainz University. "Although the system consists only of a laser pulse and is thus very small, it can—in principle—eradicate errors immediately." Thus, there is no need to generate individual photons as qubits via numerous light pulses and then have them interact as logical qubits. "We need just a single light pulse to obtain a robust logical qubit,"
A physical qubit with built-in error correction
0 notes
Text
The Impact of Quantum Computing on Future UI/UX Design Trends
The emergence of quantum computing in UI/UX designs signals a monumental shift for the computing sector. By utilizing proprietary dynamics like quantum superposition and quantum entanglement, quantum computers can analyze intricate datasets and execute calculations at unprecedented speeds compared to classical computing.
As quantum computing technology continues maturing in the next decade, it may substantially influence user interface (UI) and user experience (UX) design paradigms across industries.
More Responsive Experiences
One prospect with quantum’s superior processing capacities in UI/UX designs involves building more adaptive, contextually-aware interfaces. Quantum machine learning models could monitor user behaviors to anticipate actions, adjusting interfaces accordingly in real-time.
Use Cases
Integration Challenges
Predictive design systems adapting interfaces based on usage patterns
Significant software engineering efforts required
Conversational interfaces leveraging quantum NLP for complex voice commands
Resource/power constraints around running advanced quantum algorithms
However, while promising for enhanced experiences in UI/UX development services, developing mature quantum ML tools could take considerable time. Progress remains at early stages presently.
More Immersive Environments
Combining quantum's speed with emerging extended reality (XR) technologies may also lead to more immersive, multi-sensorial interfaces and experiences in UI/UX designs. Quantum simulations can help render intricate virtual environments by modeling molecular dynamics.
Use Cases
Deployment Challenges
Photorealistic holographic telepresence leveraging quantum avatar rendering
Significant hardware requirements for running quantum algorithms in real-time
Enhanced molecular modeling for drug discovery
Lack of talent with specialized quantum software development skills
However, most experiential concepts leveraging quantum-XR integration currently remain speculative rather than immediately implementable. Significant advances in UI/UX development services must still happen first across quantum, classical, and XR spheres before such inventions materialize commercially.
Reimagining System Design Principles
Looking beyond individual interfaces, quantum principles of mobile UI/UX designs could also inform how we philosophically conceive design systems themselves.
For example, quantum physics notions like superposition defy binary either/or logic, existing in multiple states simultaneously.
Adopting such quantum-inspired models may assist in building more dynamic, modular UI/UX design languages.
Rather than defined stylistic constraints, components could adapt on-the-fly based on user contexts, perhaps changing colors, shapes, sizes, etc. This increased fluidity and flexibility in UI/UX designs could enhance experiences, though likely works best balanced with retaining some grounded frameworks still for manageability.
Key Deployment Considerations
While an exciting topic for hypothesizing, actualizing quantum computing’s UI/UX design impacts requires overcoming ongoing platform challenges first. Current quantum computers remain highly specialized machines with limited applications beyond research realms.
Expanding the scope and scalability of quantum computing hardware/software stacks will take dedicated engineering efforts across years of continual enhancements to core components like qubits.
Quantum technologies with legacy UI/UX design infrastructure also poses complex difficulties around reconciling very different, often incompatible system architectures. Large-scale migration initiatives typically demand long phases for modifications to both quantum and classical computing spheres.
When assessing speculative use cases, it is also helpful distinguishing between quantum-inspired software design versus running applications directly on quantum processing units (QPUs). The former provides more flexibility for tangible near-term innovation in mobile UI/UX designs.
Conclusion
In summary, while actualizing the full breadth of quantum computing’s paradigm-shifting potential systemwide requires a longer-term outlook, an UI/UX design agency can start exploring quantum-inspired ideas today through prototypes and conceptual models to get ahead on future capabilities.
As quantum platforms progress, keeping abreast of the latest developments can assist designers in recognizing new opportunities early.
Contact Consagous Technologies, an award-winning UI/UX design agency, to learn about our user-centric design and engineering services. Our experts continually investigate leading-edge interfaces and experiences that may someday benefit clients on the quantum computing frontier.
Get in touch with us today!
0 notes
Text
How does quantum computing work
Introduction
In the ever-evolving landscape of technology, quantum computing stands out as a revolutionary paradigm that has the potential to reshape the way we process information. This article will delve into the intricacies of quantum computing, unraveling the mystery behind its functioning, and exploring its profound implications for the future. Let's embark on a journey to understand "How does quantum computing work?"
Understanding the Basics
At its core, quantum computing leverages the principles of quantum mechanics to perform computations. Unlike classical computers that use bits to represent either a 0 or a 1, quantum computers use quantum bits, or qubits. Qubits can exist in multiple states simultaneously, thanks to a phenomenon known as superposition.
Superposition: The Quantum Advantage
Superposition allows qubits to exist in a multitude of states at the same time, exponentially increasing the computational power of quantum computers. This ability to process multiple possibilities simultaneously opens doors to solving complex problems that were previously insurmountable for classical computers.
Entanglement: Quantum Connectivity
Another key principle of quantum mechanics is entanglement, wherein qubits become interconnected and the state of one qubit instantaneously influences the state of its entangled partner, regardless of the physical distance between them. This phenomenon enables quantum computers to perform complex computations faster and more efficiently than classical counterparts.
Quantum Gates and Circuits
Similar to classical computers that employ logic gates for processing information, quantum computers utilize quantum gates. However, quantum gates operate based on the principles of quantum mechanics, manipulating qubits through operations like superposition and entanglement. Quantum circuits are constructed by combining these gates, creating intricate pathways for quantum information processing.
Applications of Quantum Computing
Cryptography: Unbreakable Codes
One of the most significant implications of quantum computing lies in its impact on cryptography. Traditional cryptographic methods rely on the difficulty of certain mathematical problems for security. Quantum computers, however, could potentially break these codes using algorithms like Shor's algorithm, jeopardizing the security of sensitive data.
Optimization Problems: Unlocking Efficiency
Quantum computing excels in solving complex optimization problems, such as portfolio optimization and logistics planning. The ability to process numerous possibilities simultaneously allows quantum computers to find optimal solutions in a fraction of the time it would take classical computers.
Drug Discovery: Accelerating Research
The pharmaceutical industry can benefit immensely from quantum computing in drug discovery. Simulating molecular structures and interactions becomes exponentially faster with quantum computers, accelerating the pace of research and development in the quest for new medications.
Financial Services: Enhancing Risk Assessment
Quantum computing's impact on the financial services industry cannot be overstated. By rapidly processing vast amounts of data, quantum computers can enhance risk assessment models, optimize investment portfolios, and simulate market scenarios with unparalleled precision.
Challenges and Future Outlook
While the potential of quantum computing is vast, there are significant challenges to overcome, including error correction, decoherence, and scalability. Researchers are actively working on addressing these issues to unlock the full potential of quantum computing.
Conclusion
In conclusion, understanding how quantum computing works opens up a world of possibilities for solving problems that were once deemed unsolvable. From revolutionizing cryptography to accelerating scientific research, quantum computing holds the key to transformative advancements across various industries. As we stand on the precipice of a quantum revolution, the question is not merely "How does quantum computing work?" but rather, "What incredible feats can it help us achieve?" The journey into the quantum realm has just begun, and the future promises a landscape shaped by the limitless potential of quantum computing.
0 notes
Text
Microsoft’s Leap Towards Fault-Tolerant Quantum Computing with Azure Quantum
New Post has been published on https://thedigitalinsider.com/microsofts-leap-towards-fault-tolerant-quantum-computing-with-azure-quantum/
Microsoft’s Leap Towards Fault-Tolerant Quantum Computing with Azure Quantum
Quantum computing, with its promise of solving complex problems that classical computers struggle with, has been a topic of intense research and development. Microsoft, a key player in the quantum computing field, is making significant strides toward achieving fault-tolerant quantum computing at scale through its Azure Quantum platform. This article takes a closer look at these developments, explaining their significance and considering how they might shape the future of computing.
Quantum at Scale: A Necessity
In the quest to use quantum computing for solving some of the biggest challenges like climate change and medical breakthroughs, experts estimate that we would need quantum computers powered by at least one million qubits. A qubit, short for quantum bit, is the fundamental unit of information in quantum computing. Unlike classical bits that can only exist in one of two states, 0 or 1, at any given time, qubits can exist in a superposition of states. This means a qubit can be in a state representing both 0 and 1 simultaneously. Moreover, qubits can be entangled with each other, where the state of one qubit depends on the state of another qubit. This enables qubits to encode complex information and parallel processing capabilities that surpass classical computing. However, qubits need to be both stable and controllable to perform these complex calculations effectively.
Achieving this scale—developing a quantum computer with a million qubits—is an enormous challenge. Currently, managing even a few qubits requires sophisticated technology and precise control. Scaling up to a million qubits multiplies these challenges in terms of maintaining qubit stability and ensuring error-free operations across such a large scale.
The Challenge of Qubit’s Sensitivity
One of the key challenges in quantum computing is the sensitivity of qubits to errors. Even minor environmental changes can lead to errors that substantially impact the reliability of quantum computers for practical uses. Moreover, even small errors can have a big impact on the processes in quantum computing. For instance, although a fidelity rate of 99.9% seems reasonable, meaning errors happen only once in every 1,000 operations, it’s quite high for quantum computing where operations run into the million qubits to solve complex problems. This can lead to many errors that stack up, making the results less reliable.
For quantum computers to work effectively and reliably, they need to perform these operations with extreme accuracy over long periods. This requirement becomes more formidable as the system expands to manage complex calculations more effectively.
Error Correction using Logical Qubits
Enhancing the robustness against errors is critical for improving the reliability and scalability of quantum computing. Researchers are actively developing error detection and correction strategies at both the physical and logical levels of qubits. While simply boosting the fidelity of physical qubits may not fully address the issue, the use of logical qubits offers a promising path forward.
Logical qubits function like repetition codes in classical computing, where information is duplicated across multiple bits to protect against errors. However, due to the no-cloning theorem in physics, direct replication of qubits is not possible. Instead, quantum error correction spreads the state of a logical qubit across several physical qubits. This redundancy enables the detection and correction of errors in individual physical qubits, maintaining the integrity of the quantum information and greatly reducing the error rate. By forming a single logical qubit from multiple physical ones, this method introduces fault tolerance. Even if some physical qubits err, the state of the logical qubit remains intact, determined by the unaltered physical qubits. This significantly boosts the stability and reliability of quantum computers, allowing them to handle more complex and lengthy computations. However, this requires a well-thought-out system with carefully designed hardware and software to manage errors effectively.
Microsoft and Quantinuum’s Error Reduction Breakthrough
In a recent collaboration, Microsoft and Quantinuum successfully addressed the longstanding challenge of qubits’ vulnerability to errors. They accomplished this by integrating Quantinuum’s hardware system with Microsoft’s qubit-virtualization or logic qubit system, resulting in an integrated and robust system that achieved an impressive 800-fold enhancement in error handling. This integration allowed researchers to carry out 14,000 independent instances without encountering any errors. Central to this achievement is Microsoft’s qubit virtualization system, which converts physical qubits into logical qubits and executes error correction. Through this virtualization system, they were able to produce four stable logical qubits from only 30 out of Quantinuum’s 32 physical qubits, showcasing an extremely low circuit error rate of 0.00001, indicating one error per 100,000 operations.
Understanding the impact of this development becomes more apparent when we think about reducing the error rate by 800 times, which is like improving a signal by 29 dB, similar to the experience of using a high-quality noise-canceling headset. Think of the background noise on an airplane as the ambient noise from physical qubits. Just as the headset cancels noise for better music listening, the qubit-virtualization system helps reduce errors caused by physical qubits during quantum computing tasks.
The Impact Beyond Error Reduction
The collaboration between Quantinuum’s hardware and Microsoft’s qubit-virtualization systems extends beyond just reducing errors. By integrating these technologies, researchers are provided with a stable platform to develop and implement complex quantum algorithms. This development could encourage innovation in areas like material science and cryptography, and improve the accessibility of quantum computing technologies. As the platform continues to mature and becomes more accessible, it might broaden access to quantum computing, enabling more scientists and institutions to engage in advanced research.
The Bottom Line
Microsoft’s pursuit of fault-tolerant quantum computing through Azure Quantum signifies a transformative leap in computational capabilities. While the focus has been on error reduction, the integration of Quantinuum’s quantum hardware with Microsoft’s qubit-virtualization systems unveils a realm of possibilities beyond mere error mitigation. This advancement doesn’t just refine error handling; it establishes a robust foundation for exploring intricate quantum algorithms. By bridging the gap between hardware and virtualization, Microsoft empowers researchers to delve into new frontiers across scientific domains like material science and cryptography.
#000#Accessibility#Algorithms#ambient#Article#azure#Azure Quantum#background#challenge#change#classical#climate#climate change#Collaboration#computer#computers#computing#cryptography#detection#development#Developments#domains#Environmental#Fault-tolerant quantum computing#focus#Foundation#Fundamental#Future#gap#Hardware
0 notes
Text
Demystifying Quantum Computing: How Does Quantum Computing Work?
Quantum computing, a field at the intersection of physics and computer science, has garnered significant attention for its potential to revolutionize the way we process information. In this comprehensive exploration, we'll unravel the intricacies of quantum computing, answering the fundamental question: How does quantum computing work?
I. Quantum Bits (Qubits): The Building Blocks of Quantum Computing
At the heart of quantum computing lies the concept of qubits, the quantum counterpart to classical bits. Unlike classical bits, which can exist in a state of either 0 or 1, qubits can exist in multiple states simultaneously, thanks to the principles of superposition. This property enables quantum computers to process vast amounts of information in parallel, exponentially increasing their computational power.
II. Superposition and Entanglement: Quantum Phenomena at Play
Superposition and entanglement are two key quantum phenomena that distinguish quantum computing from classical computing. Superposition allows qubits to exist in multiple states at once, significantly expanding the computational possibilities. Entanglement links qubits in a way that the state of one qubit directly influences the state of another, even when separated by large distances. These phenomena form the basis of quantum parallelism and coherence, fundamental to quantum computing's power.
III. Quantum Gates: Executing Quantum Operations
Similar to classical computers, quantum computers use logic gates to perform operations. However, quantum gates operate on qubits and leverage the principles of superposition and entanglement. The most common quantum gates include Hadamard gates, CNOT gates, and phase gates. Understanding how these gates manipulate qubits is essential to comprehend the functioning of quantum algorithms.
IV. Quantum Algorithms: Harnessing Quantum Parallelism
Quantum algorithms exploit the parallelism inherent in quantum computing to solve certain problems exponentially faster than classical algorithms. Notable examples include Shor's algorithm for factoring large numbers and Grover's algorithm for unstructured search problems. These algorithms showcase the immense computational advantages that quantum computers offer for specific tasks.
V. Quantum Measurement: Extracting Information
Quantum measurement plays a crucial role in quantum computing. When a quantum system, represented by qubits, is measured, it collapses into one of the possible states. This process extracts information from the quantum system, allowing us to obtain the final output of a quantum computation. The probabilistic nature of measurement is a distinctive feature of quantum computing.
VI. Quantum Hardware: Turning Theory into Reality
Building a quantum computer requires sophisticated hardware. Quantum processors, often operating at extremely low temperatures, manipulate qubits using techniques like superconductivity or trapped ions. Quantum error correction is another critical aspect, addressing the inherent susceptibility of qubits to environmental noise and maintaining the integrity of quantum computations.
VII. Challenges and Limitations: Navigating the Quantum Landscape
Despite the promise of quantum computing, there are significant challenges and limitations. Quantum decoherence, where qubits lose their quantum properties due to interactions with the environment, poses a major obstacle. Researchers are actively working on error correction techniques and building fault-tolerant quantum computers to overcome these challenges.
VIII. Quantum Supremacy: Achieving Milestones
Quantum supremacy refers to the point at which a quantum computer outperforms the most advanced classical computers in specific tasks. Google's 2019 achievement of quantum supremacy marked a significant milestone, demonstrating that a quantum computer could solve a task faster than the most powerful supercomputers.
IX. Future Prospects: Quantum Computing on the Horizon
As researchers continue to push the boundaries of quantum computing, the future holds exciting possibilities. From advancements in quantum hardware to the development of new algorithms, the field is poised for rapid growth. Quantum computing's potential applications span fields like cryptography, optimization problems, and drug discovery.
Conclusion:
In conclusion, the workings of quantum computing are rooted in the principles of quantum mechanics, introducing concepts like superposition, entanglement, and quantum parallelism. As quantum computers evolve from theoretical concepts to practical applications, understanding the fundamentals becomes increasingly important. The journey into the quantum realm is filled with challenges, but the potential benefits for solving complex problems are vast, making quantum computing a captivating and transformative field.
0 notes