#bioelectronics
Explore tagged Tumblr posts
Text
For years, Prof. Bozhi Tian's lab has been learning how to integrate the world of electronics—rigid, metallic, bulky—with the world of the body—soft, flexible, delicate. In their latest work, they have created a prototype for what they call "living bioelectronics": a combination of living cells, gel, and electronics that can integrate with living tissue. Their study was published May 30 in Science. The patches are made of sensors, bacterial cells, and a gel made from starch and gelatin. Tests in mice found that the devices could continuously monitor and improve psoriasis-like symptoms, without irritating skin. "This is a bridge from traditional bioelectronics, which incorporates living cells as part of the therapy," said Jiuyun Shi, the co-first author of the paper and a former Ph.D. student in Tian's lab (now with Stanford University).
Continue Reading.
76 notes
·
View notes
Text

New, more biocompatible materials for bioelectronic applications
Bioelectronics is a field of research in which biology and electronics converge. In medicine, for example, an external electric current is used to cure or monitor diseases of the nervous system, and also to monitor biomarkers in situ. Devices made of conductive materials are used for these applications. The most widely used conductive polymer so far in energy and biomedical applications is PEDOT doped with PSS, known as PEDOT:PSS. Despite its exceptional properties, new conductive materials that can improve some of its limitations, such as biocompatibility, still need to be developed. A study conducted by CIC biomaGUNE's Biomolecular Nanotechnology group is proposing a mechanism for doping PEDOT using a robust engineered protein (PEDOT:Protein); the outcome is a hybrid material with ionic and electronic conductivity, which is quite similar to PEDOT:PSS in some cases. The paper is published in the journal Small.
Read more.
#Materials Science#Science#Biocompatible#Biomaterials#Electronics#Bioelectronics#Polymers#Electrical conductivity#Proteins
12 notes
·
View notes
Text

Electrical Orchestration
How hollow organoids – lab-grown 3D tissue models – can be shaped and inflated by external electrical stimulation highlights the role of life's natural bioelectrical systems (as in water regulation) and has potential for applications in fields such as bioengineering and developmental biology
Read the published research article here
Image from work by Gawoon Shim and Isaac B. Breinyn, and colleagues
Department of Mechanical and Aerospace Engineering, Princeton University, Princeton, NJ, USA
Image originally published with a Creative Commons Attribution 4.0 International (CC BY 4.0)
Published in Nature Communications, April 2024
You can also follow BPoD on Instagram, Twitter and Facebook
#science#biomedicine#immunofluorescence#biology#organoids#electrical stimulation#bioelectronics#developmental biology
8 notes
·
View notes
Text
#ElectrochemicalDevices#SensorTechnology#Bioelectronics#ElectrochemicalSensors#EnergyStorageDevices#BatteryTech#FuelCells#WearableSensors#PointOfCareDevices#ElectroanalyticalDevices#GreenEnergyTech#LabOnChip#NextGenDiagnostics
0 notes
Text
🧬 Hydrogel Semiconductors: A Revolution in Bioelectronics
The future of healthcare is here—and it’s softer, smarter, and more biocompatible than ever. Hydrogel semiconductors are breaking boundaries, merging biology with technology to power next-gen medical devices.
💡 What You’ll Discover in the Article: ✔️ How hydrogel semiconductors improve biocompatibility and flexibility ✔️ Applications in implantable devices, biosensors, and wound care ✔️ The challenges and breakthroughs in this exciting field ✔️ How this innovation is set to transform patient outcomes
✨ Why It Matters:
Hydrogel semiconductors closely mimic living tissue, reducing inflammation and improving biosensing.
They open doors to better pacemakers, brain-machine interfaces, and continuous health monitoring.
🔗 Explore the full article and see how this breakthrough is shaping the future of bioelectronics.
Let’s build a healthier tomorrow with cutting-edge technology and innovative thinking.
0 notes
Text
Bioelectronics Enter A New Era in Skin Tech
For years, Prof. Bozhi Tian’s lab has been learning how to integrate the world of electronics—rigid, metallic, bulky—with the world of the body—soft, flexible, delicate. In their latest work, they have created a prototype for what they call “living bioelectronics”: a combination of living cells, gel, and electronics that can integrate with living tissue. The patches are made of sensors,…

View On WordPress
0 notes
Text
Bioelectronics Market Is Estimated To Witness High Growth Owing To Rising Applications In Healthcare
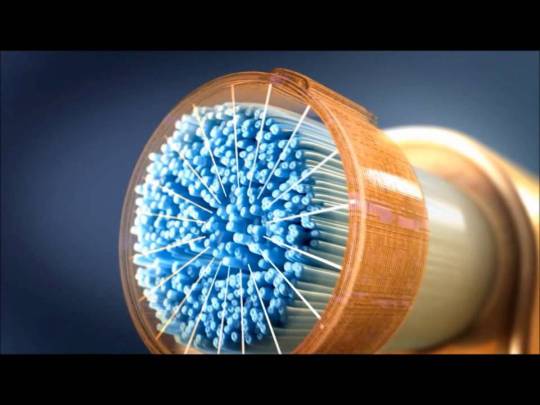
Bioelectronics is an interdisciplinary field that involves the merging of biology and electronics to develop devices and systems that combine biological and synthetic materials. Bioelectronics has applications in healthcare for developing implantable and wearable medical devices for monitoring health parameters such as electrocardiograms (ECG), electromyography (EMG), and electroencephalograms (EEG). Biosensors integrated into these devices can analyze blood, sweat or tissue samples to detect biomarkers for diseases. The global bioelectronics market is estimated to be valued at US$ 23530 Mn in 2023 and is expected to exhibit a CAGR of 11% over the forecast period 2023 to 2030, as highlighted in a new report published by Coherent Market Insights. Market Dynamics The rising applications of bioelectronics in healthcare is driving the growth of the global bioelectronics market. Bioelectronic devices aid in monitoring health parameters remotely and continuously, thereby improving disease diagnosis and management. This is expected to support market growth over the forecast period. Further, ongoing research focused on the development of advanced bioelectronic medicines is also fueling demand. For instance, researchers are working on developing electronic drug delivery systems coupled with biological components to treat diseases including diabetes, cancer, or inflammatory conditions with tailored drug release profiles. However, high costs associated with developing advanced bioelectronic systems and obtaining regulatory approvals pose a challenge to market players. SWOT Analysis Strength: Bioelectronics technology has capabilities that could positively impact and advance healthcare. It has the potential to improve disease diagnosis and monitoring through more precise and personalized detection methods. Devices incorporating bioelectronics may help enhance treatment through remote monitoring and administration of therapies. This represents a strength as patients could benefit significantly from more proactive and tailored care approaches. Weakness: The bioelectronics field is still developing and refinements are ongoing to further strengthen performance of devices and integrate biocompatible materials. This presents some uncertainties that may discourage adoption until technologies achieve more proven results at commercial scales. Obtaining regulatory approval for new classes of medical devices can be lengthy and costly as well. Opportunity: An aging global population is driving demand for advanced healthcare solutions. The medical technology industry is actively investing in bioelectronics as a means to deliver personalized care for chronic diseases and conditions. This growing preventive medicine trend presents commercial opportunities as new types of diagnostic tools and therapeutic devices are brought to markets. Biosensors also show promise for applications in food safety, environmental monitoring and national security. Threats: Competing drug and device manufacturers may attempt to delay market entry of disruptive new bioelectronics through regulatory and legal challenges. Strict rules govern biosensing technologies and any adverse events involving new products could damage brands. Rapid changes occurring as the field evolves also present technical threats if standards and interfaces are not adequately stabilized before broad adoption. Key Takeaways The global bioelectronics market forecast is expected to witness high growth over the forecast period of 2023 to 2030 supported by a growing aging population with chronic conditions seeking more advanced healthcare solutions. The global bioelectronics market is estimated to be valued at US$ 23530 Mn in 2023 and is expected to exhibit a CAGR of 11% over the forecast period 2023 to 2030.
Regional analysis: The Asia Pacific region is dominating the global bioelectronics market with the highest growth rate over the forecast period. This can be attributed to factors such as rising medical expenditures, expanding biotechnology industries, and government initiatives and investments promoting development of indigenous bioelectronic technologies particularly in India, China, and South Korea. The large patient pools and growing middle-class populations seeking access to advanced healthcare in Asia Pacific economies also contribute to high market potential.
Key players operating in the bioelectronics market are BBI-Biotech GmbH Bioengineering AG, Danaher Corporation, Eppendorf AG, Getinge, Infors HT, Merck KGAA, Sartorius AG, Solaris Biotech Solutions, and Thermo Fisher Scientific, Inc. Leading players are focused on new product approvals, partnerships and acquisitions to enhance their product portfolio and geographic presence in this rapidly evolving industry.
Get more insights on this topic: https://www.newsstatix.com/bioelectronics-market-industry-insights-trends-bioelectronics-market/
Explore more information on this topic, Please visit: https://www.urdughr.com/2023/12/recycled-construction-aggregates-a-sustainable-solution-for-growing-construction-needs.html
#Bioelectronics#Bioelectronics Market#Bioelectronics Market size#Bioelectronics Market share#Bioelectronics Market demand#Bioelectronics Market analysis#Bioelectronics Market scope
0 notes
Text
#bioelectronicsandbiosensors#biosensors#bioelectronics#sensortechnology#bioelectronicsandbiosensorsmarketreport#bioelectronicsandbiosensorsmarketsize#bioelectronicsandbiosensorsmarketshare#bioelectronicsandbiosensorsmarketgrowth#bioelectronicsandbiosensorsmarketanalysis#grandresearchstore
0 notes
Text
The scientific community has long been enamored of the potential for soft bioelectronic devices. But they’ve faced hurdles in identifying materials that are biocompatible and have all of the necessary characteristics to operate effectively. The new research is a step in the right direction. Researchers have modified an existing biocompatible material so that it conducts electricity efficiently in wet environments and can send and receive ionic signals from biological media.
Continue Reading.
49 notes
·
View notes
Text
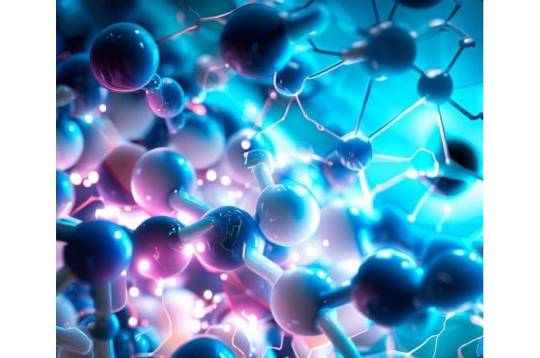
New study challenges conventional understanding of charging process in electrochemical devices
A new study by researchers at the University of Cambridge reveals a surprising discovery that could transform the future of electrochemical devices. The findings offer new opportunities for the development of advanced materials and improved performance in fields such as energy storage, brain-like computing, and bioelectronics. Electrochemical devices rely on the movement of charged particles, both ions and electrons, to function properly. However, understanding how these charged particles move together has presented a significant challenge, hindering progress in creating new materials for these devices. In the rapidly evolving field of bioelectronics, soft conductive materials known as conjugated polymers are used for developing medical devices that can be used outside of traditional clinical settings. For example, this type of materials can be used to make wearable sensors that monitor patients' health remotely or implantable devices that actively treat disease. The greatest benefit of using conjugated polymer electrodes for this kind of devices is their ability to seamlessly couple ions, responsible for electrical signals in the brain and body, with electrons, the carriers of electrical signals in electronic devices. This synergy improves the connection between the brain and medical devices, effectively translating between these two types of signals.
Read more.
#Materials Science#Science#Electrochemistry#Bioelectronics#Conjugated polymers#Polymers#University of Cambridge
11 notes
·
View notes
Text
Tiny magnetic discs offer remote brain stimulation without transgenes
New Post has been published on https://thedigitalinsider.com/tiny-magnetic-discs-offer-remote-brain-stimulation-without-transgenes/
Tiny magnetic discs offer remote brain stimulation without transgenes


Novel magnetic nanodiscs could provide a much less invasive way of stimulating parts of the brain, paving the way for stimulation therapies without implants or genetic modification, MIT researchers report.
The scientists envision that the tiny discs, which are about 250 nanometers across (about 1/500 the width of a human hair), would be injected directly into the desired location in the brain. From there, they could be activated at any time simply by applying a magnetic field outside the body. The new particles could quickly find applications in biomedical research, and eventually, after sufficient testing, might be applied to clinical uses.
The development of these nanoparticles is described in the journal Nature Nanotechnology, in a paper by Polina Anikeeva, a professor in MIT’s departments of Materials Science and Engineering and Brain and Cognitive Sciences, graduate student Ye Ji Kim, and 17 others at MIT and in Germany.
Deep brain stimulation (DBS) is a common clinical procedure that uses electrodes implanted in the target brain regions to treat symptoms of neurological and psychiatric conditions such as Parkinson’s disease and obsessive-compulsive disorder. Despite its efficacy, the surgical difficulty and clinical complications associated with DBS limit the number of cases where such an invasive procedure is warranted. The new nanodiscs could provide a much more benign way of achieving the same results.
Over the past decade other implant-free methods of producing brain stimulation have been developed. However, these approaches were often limited by their spatial resolution or ability to target deep regions. For the past decade, Anikeeva’s Bioelectronics group as well as others in the field used magnetic nanomaterials to transduce remote magnetic signals into brain stimulation. However, these magnetic methods relied on genetic modifications and can’t be used in humans.
Since all nerve cells are sensitive to electrical signals, Kim, a graduate student in Anikeeva’s group, hypothesized that a magnetoelectric nanomaterial that can efficiently convert magnetization into electrical potential could offer a path toward remote magnetic brain stimulation. Creating a nanoscale magnetoelectric material was, however, a formidable challenge.
Kim synthesized novel magnetoelectric nanodiscs and collaborated with Noah Kent, a postdoc in Anikeeva’s lab with a background in physics who is a second author of the study, to understand the properties of these particles.
The structure of the new nanodiscs consists of a two-layer magnetic core and a piezoelectric shell. The magnetic core is magnetostrictive, which means it changes shape when magnetized. This deformation then induces strain in the piezoelectric shell which produces a varying electrical polarization. Through the combination of the two effects, these composite particles can deliver electrical pulses to neurons when exposed to magnetic fields.
One key to the discs’ effectiveness is their disc shape. Previous attempts to use magnetic nanoparticles had used spherical particles, but the magnetoelectric effect was very weak, says Kim. This anisotropy enhances magnetostriction by over a 1000-fold, adds Kent.
The team first added their nanodiscs to cultured neurons, which allowed then to activate these cells on demand with short pulses of magnetic field. This stimulation did not require any genetic modification.
They then injected small droplets of magnetoelectric nanodiscs solution into specific regions of the brains of mice. Then, simply turning on a relatively weak electromagnet nearby triggered the particles to release a tiny jolt of electricity in that brain region. The stimulation could be switched on and off remotely by the switching of the electromagnet. That electrical stimulation “had an impact on neuron activity and on behavior,” Kim says.
The team found that the magnetoelectric nanodiscs could stimulate a deep brain region, the ventral tegmental area, that is associated with feelings of reward.
The team also stimulated another brain area, the subthalamic nucleus, associated with motor control. “This is the region where electrodes typically get implanted to manage Parkinson’s disease,” Kim explains. The researchers were able to successfully demonstrate the modulation of motor control through the particles. Specifically, by injecting nanodiscs only in one hemisphere, the researchers could induce rotations in healthy mice by applying magnetic field.
The nanodiscs could trigger the neuronal activity comparable with conventional implanted electrodes delivering mild electrical stimulation. The authors achieved subsecond temporal precision for neural stimulation with their method yet observed significantly reduced foreign body responses as compared to the electrodes, potentially allowing for even safer deep brain stimulation.
The multilayered chemical composition and physical shape and size of the new multilayered nanodiscs is what made precise stimulation possible.
While the researchers successfully increased the magnetostrictive effect, the second part of the process, converting the magnetic effect into an electrical output, still needs more work, Anikeeva says. While the magnetic response was a thousand times greater, the conversion to an electric impulse was only four times greater than with conventional spherical particles.
“This massive enhancement of a thousand times didn’t completely translate into the magnetoelectric enhancement,” says Kim. “That’s where a lot of the future work will be focused, on making sure that the thousand times amplification in magnetostriction can be converted into a thousand times amplification in the magnetoelectric coupling.”
What the team found, in terms of the way the particles’ shapes affects their magnetostriction, was quite unexpected. “It’s kind of a new thing that just appeared when we tried to figure out why these particles worked so well,” says Kent.
Anikeeva adds: “Yes, it’s a record-breaking particle, but it’s not as record-breaking as it could be.” That remains a topic for further work, but the team has ideas about how to make further progress.
While these nanodiscs could in principle already be applied to basic research using animal models, to translate them to clinical use in humans would require several more steps, including large-scale safety studies, “which is something academic researchers are not necessarily most well-positioned to do,” Anikeeva says. “When we find that these particles are really useful in a particular clinical context, then we imagine that there will be a pathway for them to undergo more rigorous large animal safety studies.”
The team included researchers affiliated with MIT’s departments of Materials Science and Engineering, Electrical Engineering and Computer Science, Chemistry, and Brain and Cognitive Sciences; the Research Laboratory of Electronics; the McGovern Institute for Brain Research; and the Koch Institute for Integrative Cancer Research; and from the Friedrich-Alexander University of Erlangen, Germany. The work was supported, in part, by the National Institutes of Health, the National Center for Complementary and Integrative Health, the National Institute for Neurological Disorders and Stroke, the McGovern Institute for Brain Research, and the K. Lisa Yang and Hock E. Tan Center for Molecular Therapeutics in Neuroscience.
#250#applications#author#background#Behavior#bioelectronics#Brain#Brain and cognitive sciences#brain research#brain stimulation#brains#Cancer#Cells#challenge#chemical#chemistry#Composition#computer#Computer Science#development#devices#Disease#disorders#DMSE#droplets#effects#electrical stimulation#electricity#electrodes#Electronics
0 notes
Text
The Spark Within: How Electricity Powers the Human Body.
Sanjay Kumar Mohindroo Sanjay Kumar Mohindroo. skm.stayingalive.in Explore how bioelectricity fuels nerves, muscles, heart rhythm, healing, and next‑gen therapies. #bioelectricity #healthtech Lighting the Hidden Power Understanding bioelectricity in life
Our bodies hum with tiny electric signals that make life possible. #bioelectricity These signals arise when charged ions move across cell…
#Action Potential#biochemistry#Bioelectricity#Bioelectronic Medicine#Cardiac Electrophysiology#healing#health#Membrane Potential#Muscle Contraction#Nerve Impulse#News#nutrition#Sanjay Kumar Mohindroo#wellness#Wound Healing
0 notes
Text
SciTech Chronicles. . . . . . . . .April 1st, 2025
#bioproducts#waste#polyhydroxyalkanoates#3D-Printing#Bellingshausen#A-84#seafloor#sediment#PEDOT:PSS#crosslinker#ions#bioelectronic#polyfluoroalkyl#graphene#FJH#GAC#renewables#topology#forecasting#spatio-temporal
0 notes
Text
Bioelectronic Systems: A Novel Approach in Controlled Drug Delivery

Abstract
Electronic drug delivery systems (EDDS) are an interesting advancement in drug delivery technology. They are portable, interactive, wirelessly networked, and enable patient-administered medication, which lowers overall healthcare costs. Controlled DDS maintains drug plasma levels constantly by releasing the definite dose of the drug at each time point for a predetermined duration. This helps in reducing the dose and dosing frequency and improves patient compliance. Lesser drug exposure to the biological environment reduces drug toxicity and adverse effects. Among controlled release. Transdermal delivery mode (referred to as patches) is more preferably used among them because of great patient compliance. Bioelectronic systems play a crucial role in electronically controlled drug delivery systems by integrating electronic components with biological systems to deliver drugs with precision and efficiency. Their efficiency is further increased when integrated into remotely operated systems. One of the main motivations for developing EDDS was to increase patient adherence to recommended drug regimens. Moreover, EDDS have demonstrated the ability to administer drugs to specific body locations on demand. This review concentrates on electronic medication delivery systems, despite the fact that there are many different types of drug delivery devices on the market. Along with their mechanism of actions are also discussed.
Introduction
Controlled drug delivery system This is the drug delivery system in which a constant level of a drug is maintained in blood and tissue for an extended period. Controlled DDS maintains drug plasma levels constantly by releasing the definite dose of the drug at each time point for a predetermined duration (Tekade et al., 2018). This helps in reducing the dose and dosing frequency and improves patient compliance. Lesser drug exposure to the biological environment reduces drug toxicity and adverse effects.
Evolution of the controlled release dosage forms First-generation: This generation of dosage forms mainly involves four types of mechanisms for drug release, which include the oral and transdermal formulations. The mechanisms involved are dissolution, osmosis, diffusion, and ion exchange. Diffusion and dissolution-controlled systems are the most widely used mechanisms of drug delivery. The success of the first generation of drugs is mainly the development of the oral and transdermal routes (Park et al., 2014).
Second-generation: These are not widely used. Electrically delivery systems were developed for introducing insulin. Due to its lesser bioavailability, it is administered many times higher per dose than is required, which results in toxicity. In the last decade of the second generation, nanoparticles that target genes and tumors were studied.
The third generation: involves the delivery of poorly water-soluble drugs, long-term and non-invasive technology for delivering proteins/nucleic acids/peptides, and drug delivery to the targeted site using nanoparticles (Yun et al., 2015).
Formulations of controlled-release medication Oral, intravenous, and transdermal patches are easily developed. Among controlled release, transdermal delivery mode (referred to as patches) is more preferably used among them because of great patient compliance.
Source : Bioelectronic Systems: A Novel Approach in Controlled Drug Delivery | InformativeBD
0 notes
Text
youtube
#Bioelectronics hydrogels#cardiac treatment#brain health#implantable devices#medical innovation#neural stimulation#heart health technology#chronic disease treatment#biocompatible materials#brain disease therapy#cardiac monitoring#drug delivery systems#neurotechnology#advanced therapies#biomedical research#healthcare innovation#hydrogel applications#medical implants#neuroprotection#smart medical technology.#Youtube
0 notes