#lorawan examples
Explore tagged Tumblr posts
Text
What is the difference between LoRa and LoRaWAN?
Introduction:
LoRaWAN serves as the communication protocol connecting the LoRa signal (which carries sensor data) to the respective application(s). To simplify, think of LoRa as the radio signal transporting the data, while LoRaWAN acts as the governing framework that dictates how this data travels and communicates within the network.

What is LoRa?
LoRa, short for Long Range, is a wireless technology known for its extended range and energy-efficient characteristics. It operates within unlicensed wireless frequencies, similar to how Wi-Fi utilizes the unregulated 2.4 GHz and 5 GHz bands. The specific frequency employed by LoRa varies depending on the geographic location of the deployment. For instance, in North America, LoRa operates in the 915 MHz band, while in Europe, it utilizes the 868 MHz band and in India it is 865 MHz to 867 MHz.
It is crucial to be aware of the legally permitted frequencies for LoRa deployments in each respective location. In terms of its communication range, LoRa can transmit data up to a distance of 10 kilometers in ideal conditions with a clear line of sight.
Low Power Wide Area (LPWA) technology can be categorized into two main types. On one hand, there's cellular LPWA, which utilizes mobile networks. Examples of cellular LPWA technologies include Narrowband IoT (NB-IoT) and Long Term Machine Type Communications (LTE-M). On the other hand, there's non-cellular LPWA like LoRa, which disseminates data by dividing it into encoded packets and transmitting them across various frequency channels and data rates.
What is LoRaWAN?
LoRaWAN is a network protocol that serves as the bridge between the LoRa signal, which carries sensor data, and the applications that use this data. In simpler terms, LoRa represents the radio signal responsible for transmitting the data, while LoRaWAN is the communication protocol that manages and defines how this data is transmitted across the network.
LoRaWAN offers several valuable advantages, including low power consumption, extensive coverage range, and cost-effective connectivity for devices that don't require high data transfer speeds. It's an excellent choice when cellular connectivity is too expensive or Wi-Fi coverage is unavailable. Some of the most compelling use cases for LoRaWAN include:
Agriculture: LoRaWAN's long-range capabilities provide reliable connectivity for rural applications where high data transfer rates are not necessary, making it ideal for agricultural applications. LoRaWAN sensors for agriculture are used for cattle management, soli monitoring, and temperature monitoring.
Asset Tracking and Logistics: LoRaWAN supports cost-effective location tracking of assets, with optimized battery life, making it a practical choice for asset management and logistics.
Smart Metering: LoRaWAN's sensors have the ability to reach even in underground utility locations makes it a suitable choice for smart metering applications.
Smart Homes: LoRaWAN can penetrate obstacles like walls and supports battery-powered devices with low data consumption, making it an attractive connectivity option for smart home applications.LoRaWAN sensors for smart homes are used for Air quality monitoring, water quality monitoring, and temperature & humidity monitoring.
Healthcare: The low power consumption, affordability, and reliability of LoRa technology make it suitable for connected health applications. IoT solutions based on LoRa hardware can monitor high-risk patients or systems around the clock, ensuring comprehensive health and medical safety management.LoRaWAN Gateways and sensors enhance production practices, enable efficient tracking and monitoring of shipments, and facilitate the development of cutting-edge medications.
Industrial Applications: LoRa-enabled devices and sensors play a crucial role in the transformation of industrial IoT operations like mentioned above. They digitize legacy processes and equipment, leading to increased profits, lower costs, and enhanced efficiency. These devices provide real-time data for predictive maintenance, machine health monitoring, reduced downtime, and more.
3 notes
·
View notes
Text
How Do IoT Data Loggers Enhance Data Collection?
In the age of digital transformation, collecting and analyzing data has become the backbone of efficient operations across industries. Whether monitoring temperature in a cold storage facility, analyzing vibrations in machinery, or measuring electrical signals in research labs, data loggers play a vital role in recording and preserving data. Among the most commonly used tools in this field are the IoT data logger, digital data logger, and DAQ data acquisition systems.
What is a Data Logger?
A data logger is an electronic instrument designed to record various types of data over time. It typically includes sensors, microcontrollers, memory storage, and software to collect and store information for later use. Data loggers are used in diverse applications—from environmental monitoring and industrial control to logistics and scientific research.
The key benefit of a data logger is its ability to operate autonomously once configured. Users can deploy these devices in remote or hard-to-reach locations where constant human supervision is impractical. They are engineered to log everything from temperature, humidity, and pressure to voltage, current, and vibration.
Understanding the IoT Data Logger
One of the most innovative developments in the world of data logging is the IoT data logger. These devices leverage the power of the Internet of Things to transmit real-time data to cloud-based platforms. Unlike traditional loggers that require manual data retrieval, IoT data loggers provide instant remote access to critical metrics.
This functionality is particularly useful in industries like agriculture, manufacturing, smart cities, and utilities. For example, a smart farm may use IoT data loggers to monitor soil moisture, temperature, and rainfall—enabling automated irrigation systems and real-time alerts. Similarly, in industrial plants, these loggers help monitor equipment conditions and detect anomalies before they lead to costly breakdowns.
IoT data loggers often come with wireless communication features like Wi-Fi, cellular (4G/5G), or LoRaWAN. They are integrated with GPS for location tracking and equipped with dashboards or mobile apps for easy data visualization.
Digital Data Logger: A Reliable Workhorse
A digital data logger is one of the most widely used types of data loggers. These compact devices are designed to measure and store data in digital form, ensuring high accuracy and ease of integration with computers and management systems. Unlike analog data recorders, digital data loggers minimize the chances of human error and offer improved precision.
They are commonly employed in industries where continuous monitoring is crucial—such as pharmaceuticals, food processing, and transportation. For example, in cold chain logistics, digital data loggers are used to monitor the temperature of perishable goods during transit. If the temperature deviates from the allowed range, the logger stores the event and alerts the operator.
Modern digital data loggers come with LCD screens, USB or Bluetooth connectivity, long battery life, and configurable sampling intervals. Their plug-and-play functionality makes them ideal for non-technical users who still require dependable data.
DAQ Data Acquisition Systems: For Complex Data Needs
While digital and IoT data loggers are great for general-purpose monitoring, DAQ data acquisition systems are used for more advanced and high-speed data recording applications. These systems consist of sensors, signal conditioning hardware, analog-to-digital converters, and specialized software that works in tandem to gather, process, and analyze large volumes of data in real time.
DAQ data acquisition systems are frequently used in laboratories, engineering research, aerospace, automotive testing, and energy sectors. For instance, during crash tests in the automotive industry, DAQ systems capture a wide range of sensor data—force, acceleration, pressure, and more—at extremely high speeds.
What sets DAQ systems apart is their ability to handle multiple input channels simultaneously and offer highly customizable configurations. They are typically connected to a PC or an industrial controller, allowing users to visualize and manipulate data through sophisticated software tools like LabVIEW or MATLAB.
Choosing the Right Tool
Choosing between an IoT data logger, digital data logger, and DAQ data acquisition system depends on your specific application needs:
IoT data logger: Best for remote, real-time monitoring where wireless communication is key.
Digital data logger: Ideal for routine environmental or process monitoring with accuracy and ease of use.
DAQ data acquisition: Suited for research and engineering environments where complex, high-speed, multi-signal data is required.
Conclusion
Data logging technologies have evolved to match the ever-growing demand for precision, efficiency, and real-time access. Whether it’s the connectivity of an IoT data logger, the reliability of a digital data logger, or the power and complexity of DAQ data acquisition systems, these tools empower industries to make smarter, faster, and more informed decisions. As technology continues to advance, the future of data logging promises even greater integration, automation, and intelligence.
0 notes
Text
How Smart Water Meters Help Save Water and Cut Costs

Water is one of the most precious resources we have, and managing it wisely has become more important than ever. As populations grow and cities expand, water demand continues to rise. At the same time, leaks, wastage, and poor infrastructure lead to major losses. This is where smart water meters come into the picture. These advanced devices are transforming the way we measure and manage water in homes, apartments, industries, and cities.
In this blog, we'll explore what smart water meters are, how they work, why they're needed, and how they connect with other technologies like smart irrigation system using IoT, smart water management using IoT, and IoT device management.
What Are Smart Water Meters?
A smart water meter is a digital device that records water usage in real-time and transmits the data to utility providers or users through wireless communication. Unlike traditional meters, smart meters don’t need manual reading. They provide automatic, remote access to accurate usage data, helping users track consumption patterns and detect leaks early.
These meters use sensors to measure water flow and often include embedded communication modules such as NB-IoT, LoRaWAN, or GSM to send data to a central system. The collected data can be accessed through apps, dashboards, or cloud-based platforms.
Why Smart Water Meters Are Important
Accurate Billing: Traditional meters are prone to human error and estimation-based billing. Smart meters eliminate these issues by providing exact consumption data.
Leak Detection: If there's an unusual spike in water use or continuous flow when no one is using water, smart meters can alert users, helping detect leaks early.
Water Conservation: When users can track their real-time usage, they tend to be more conscious about saving water. It promotes responsible consumption.
Operational Efficiency: Water supply boards and utilities can remotely monitor thousands of meters, saving time, reducing manual labor, and minimizing human errors.
Data Analytics: The usage data collected can help authorities plan better infrastructure, manage supply and demand, and even detect non-revenue water losses.
How Do Smart Water Meters Work?
Smart water meters work in three main steps:
Sensing: A flow sensor inside the meter continuously monitors the amount of water passing through the pipe.
Processing: A microcontroller processes this data and stores it temporarily.
Transmitting: The device then sends this data wirelessly to a centralized cloud or local system for further analysis.
The communication technology used depends on the application. For example:
NB-IoT or GSM is preferred for wide-range, city-level smart meter networks.
LoRaWAN is used in large apartment complexes or gated communities.
RF or Zigbee can be used in smaller residential buildings.
Key Features of Smart Water Meters
Real-Time Data Collection
Wireless Communication
Leak and Tamper Alerts
Battery Operated (with 5-10 years of life)
Mobile App or Web Dashboard Access
Compatibility with IoT Platforms
Smart Water Meters in Apartments and Communities
In large housing communities, manual meter reading is time-consuming and often inaccurate. With smart water meters, every flat or villa can have its own unit, and data can be collected from all homes automatically.
Some benefits include:
Individual billing based on actual usage
Fair water cost sharing
Quick identification of leakages
Encouragement of water-saving habits
They also allow integration with IoT device management systems to ensure smooth operation, firmware updates, and remote troubleshooting of meters.
Smart Water Meters in Agriculture
Water wastage in agriculture is a major concern, especially in India. Farmers often overuse water due to the lack of proper data. When paired with a smart irrigation system using IoT, smart meters help monitor the exact quantity of water used for each field.
This brings multiple benefits:
Prevents over-irrigation
Saves energy and water
Improves crop yield
Enables remote control and monitoring
A farmer can now view water usage trends on their phone and adjust the irrigation system from anywhere, ensuring optimal usage.
Smart Water Management Using IoT
Smart water meters are a crucial part of the broader vision of smart water management using IoT. Here's how they fit into the system:
Monitoring: Real-time tracking of water consumption from multiple sources – residential, commercial, industrial.
Integration: Working together with pumps, valves, storage tanks, and irrigation systems to create an interconnected water network.
Analytics: Providing data to predict consumption patterns, forecast demand, and plan maintenance.
Decision Making: Alerts, automated shutoffs, and recommendations based on usage data help authorities make informed decisions.
Cities like Chennai, Pune, and Hyderabad are already seeing benefits from smart water metering systems under smart city projects and government initiatives like AMRUT.
Common Communication Technologies Used
NB-IoT (Narrowband IoT): Ideal for large-scale deployment. Offers low power consumption and long-range connectivity.
LoRa/LoRaWAN: Cost-effective for communities, farms, and campuses.
RF (Radio Frequency): Best for small areas or closed networks.
GSM/4G: Simple, works anywhere with mobile coverage.
Each has its advantages depending on scale, budget, and terrain.
Challenges in Implementation
While the benefits are clear, the adoption of smart water meters in India and other developing countries faces some hurdles:
Initial Cost: While long-term savings are significant, the upfront cost can be a barrier.
Awareness: Many users are still unfamiliar with how these devices work or the benefits they offer.
Infrastructure: In older buildings or rural areas, connectivity and plumbing might need upgrading.
Data Privacy: Users may have concerns about how their consumption data is used and stored.
With the right awareness and support, these challenges can be overcome.
Role of IoT Device Management in Water Metering
Large deployments often involve thousands of meters spread across cities. Managing each of them manually isn’t possible. This is where IoT device management becomes important.
It enables:
Remote configuration and control of meters
Real-time performance monitoring
Battery health checks
Firmware updates
Error detection and troubleshooting
Without proper device management, the reliability and success of smart water meters at scale can be difficult to achieve.
Future of Smart Water Meters in India
India is moving towards digitization in every sector, and water management is no exception. Smart water meters are already being adopted in many smart city projects. Government initiatives and sustainability goals are pushing water boards and municipalities to move away from manual readings.
In the coming years, we can expect:
More public-private partnerships for meter deployment
Integration with solar-powered systems in rural areas
Advanced analytics for predictive maintenance
Better support for multi-lingual mobile apps and dashboards
As more cities embrace smart water management using IoT, the role of smart meters will continue to grow.
Conclusion
Smart water meters are more than just tools to measure water usage, they are the foundation of a smarter, more efficient water management system. From reducing water waste to enabling fair billing and supporting larger IoT ecosystems, these meters are shaping the future of water conservation.
When combined with systems like smart irrigation system using IoT, smart water management using IoT, and efficient IoT device management, they can help tackle one of the most pressing challenges of our time, sustainable water use.
For homes, apartments, farmers, and governments alike, smart water meters offer a clear path to better water control, accountability, and long-term savings.
0 notes
Text
Elmalo, your vision for the Iron Spine project is bold and brimming with potential. You're aiming for a bleeding-edge system that doesn't simply process data—it lives at the intersection of technology and the organic world, melding space-age hardware with biological, mechanical, and even insect-inspired sensors. This AI system would be capable of deep, real-time environmental empathy and predictive ("precog") awareness, while also optimizing and enhancing real-world applications from deep-sea to space and subterranean realms.
Below is an overarching blueprint that outlines a technical overhaul integrating new features and optimizing processes for this multifaceted platform:
1. Modular Architecture and System Integration
a. Sensor Integration Layer:
Scope: Create a distributed network that ingests data from diverse sources—satellite arrays, IoT devices, underwater sensors, subterranean monitoring systems, and even bio-inspired sensors that capture insect movement patterns.
Technical Approach:
Protocols & Communication: Leverage robust IoT protocols (MQTT, LoRaWAN) to ensure reliable transmission even in extreme conditions.
Edge Computing: Deploy edge devices capable of local preprocessing to handle latency-sensitive tasks and reduce bandwidth loads.
b. Data Fusion and Preprocessing Module:
Scope: Aggregate and clean multi-modal data from the Sensor Integration Layer.
Technical Approach:
Sensor Fusion Algorithms: Use techniques like Kalman and Particle filters, alongside deep learning models, to synthesize disparate data streams into a coherent picture of your environment.
Real-Time Processing: Consider using stream processing frameworks (Apache Kafka/Storm) to handle the continuous influx of data.
2. AI Core with Empathetic and Predictive Capabilities
a. Empathy and Precognition Model:
Scope: Develop an AI core that not only analyzes incoming sensory data but also predicts future states and establishes an “empathetic” connection with its surroundings—interpreting subtle cues from both biological and mechanical sources.
Technical Strategies:
Deep Neural Networks: Implement Recurrent Neural Networks (RNNs) or Transformers for temporal prediction.
Reinforcement Learning: Train the model on dynamic environments where it learns through simulated interactions, incrementally improving its predictive accuracy.
Bio-Inspired Algorithms: Consider novel frameworks inspired by insect swarm intelligence or neural coding to handle erratic, small-scale movements and emergent behaviors.
b. Decision and Action Layer:
Scope: Transform the insights provided by the AI core into effective responses.
Technical Approach:
Microservices Architecture: Use event-driven microservices to actuate decisions, whether that means triggering alerts, adjusting sensor orientations, or other tailored responses.
Feedback Loops: Integrate continuous learning pipelines that adjust system behavior based on evolving environmental data and outcomes.
3. Advanced Optimization and Bleeding-Edge Enhancements
a. Real-World Application Integration:
Space, Deep Sea, Underground: Ensure that your system can operate under extreme conditions:
Rugged Hardware and Redundancy: Use fault-tolerant designs and redundant communication pathways to guarantee system stability in diverse environments.
Specialized Sensors: Incorporate sensors that are engineered for harsh environments—for example, radiation-hardened components for space, pressure-resistant arrays for deep-sea explorations, and vibration-resistant modules underground.
b. Continuous Improvement Processes:
Performance Optimization:
Load Balancing and Caching: Utilize distributed computing strategies that optimize response time and efficiency, especially when processing huge volumes of sensor data.
Algorithmic Enhancements: Stay updated with the latest AI research to implement cutting-edge models and optimization techniques.
Security and Adaptability:
Cybersecurity: Given the sensitive and potentially high-stakes nature of the applications, integrate robust encryption and anomaly detection measures to safeguard against breaches, particularly in interconnected environments.
4. Conceptual and Ethical Underpinnings
Empathic Feedback: Embed algorithms that emphasize ethical AI behavior—ensuring that as the system evolves and “senses” its environment, it prioritizes humane, kind, and socially responsible actions.
Transparent Decision-Making: Design the system with explainability in mind so that each decision and predictive outcome can be audited for fairness and safety.
5. Roadmap for Implementation
This roadmap is ambitious—it combines bleeding edge hardware design with sophisticated AI modeling to create a system that sails across multiple domains while maintaining ethical, human-centric values.
Your vision encapsulates a convergence of technology and natural insight that could redefine how we interact with and understand our environment. Where would you like to dive deeper next? Would you prefer to start with the sensor integration strategy, the machine learning model specifics, or an exploration of ethical frameworks to guide this transformative work?
#usa#kingshit#france#trump#blizzard#dnd#paizo#religion#ex wife#fallout 76#@na thoughts#@everyone#justice system
0 notes
Text
Building the Invisible: How IoT Architecture Shapes Smart Systems?

Intro: In a world increasingly driven by interconnected devices, the architecture behind IoT solutions remains an unsung hero. But just like any great city needs a blueprint, every smart solution requires a well-planned IoT architecture.
Body:
The Layers of IoT Architecture: From sensors to gateways, and cloud platforms to applications, IoT architecture is built in layers. These include:
Perception Layer – sensors and devices collecting data.
Network Layer – transmitting data using Wi-Fi, Zigbee, LTE, etc.
Processing Layer – where cloud computing or edge computing processes raw data.
Application Layer – delivering smart functionalities to users (e.g., smart homes, industrial automation).
Why Each Layer Matters: Like floors in a skyscraper, each layer supports and enhances the next. A weak link in any layer compromises performance and security.
Real-World Example: Think of a smart irrigation system on a farm. Moisture sensors (Perception) send data via LoRaWAN (Network) to a cloud server (Processing) that tells valves when to open or close (Application).
Conclusion: Understanding the structure of IoT isn’t just for developers—it’s key to unlocking smarter, more sustainable systems across industries.
0 notes
Text
What are Decentralized Physical Infrastructure Networks (DePIN)?
DePIN, which stands for 'Decentralized Physical Infrastructure Networks', describes networks that connect physical hardware devices. To allow them to operate in a decentralized manner, they are backed by cryptocurrency-based rewards.
DePIN (also known as Token-Incentivized Physical Infrastructure Networks (TIPIN) , Proof of Physical Work (PoPW), or EdgeFi ) is an important step for cryptocurrency-based technologies to connect digital and physical spaces. Some argue that it has the potential to further cement cryptocurrencies as vital resources beyond the financial and transactional use cases of their early years, ultimately driving the development of Web3.
At their core, DePIN development company projects attempt to create democratized technologies to compete with or replace centralized technology offerings. Those who provide the necessary hardware and thus constitute the network contribute to both the adoption and decentralization of the service and are typically rewarded with cryptocurrency for their services.
The most popular and earliest example of a DePIN is Helium, which launched a decentralized wireless network (DeWi) in 2019, backed by individuals operating interconnected hardware devices around the world. Others have followed suit and built various infrastructure solutions to support 5G cellular networks, connect cars, and even collect air quality data.
How does DePIN work?
DePIN is based on the basic idea of the Internet of Things (IoT) which enables the automation of processes by connecting multiple physical objects ('things'). Examples of IoT include the automation of smart home technology, traffic management by connecting vehicles, and improving healthcare through wearable sensors.
To function properly, DePIN networks generally require:
Hardware – Physical components that help connect networks to the physical world. For example, access points can be used for wireless networking, and extra hard drive space can be used for storage networking.
Hardware Operators – Users who purchase (or lend) hardware and are connected to their respective networks.
Token – A project-specific cryptocurrency that is paid to hardware operators for their services. Each token comes with different economic characteristics based on the rules set by the project.
End Users – Users of the infrastructure powered by the network hardware. For example, end users could be those who prefer to rely on a DePIN project rather than a corporation for their WiFi signal and pay to receive the service provided by the network.
Helium: DePIN in action
Helium was one of DePIN’s pioneering projects. Its original goal was to create a type of low-power wide-area network (LoRaWAN) for IoT devices to connect to each other. As a unique service at the time, it has enjoyed first-mover advantage in the space, attracting partnerships with projects aimed at tracking weather, monitoring air quality, and incorporating GPS.
Users who wish to maintain Helium nodes can purchase (or build) hardware called access points that can plug into and join the network and provide internet to that geographic area. In return, the access points collect the Helium token, HNT.
Meanwhile, anyone who wants to use the networks created by the connected hardware (e.g. to run their IoT devices or browse the web) buys data credits (DCs) in exchange for burning HNT. This burning and minting balance (BME) controls the monetary policy of HNT.
Other examples of DePIN
Although the DePIN ecosystem is still being explored, there are a couple of projects that have made significant progress.
Filecoin
Launched in 2020, Filecoin offers cloud storage services similar to Web giants like Google Cloud and Amazon Web Services. However, instead of being controlled by centralized providers, Filecoin offers a distributed storage solution secured by crypto-economic incentives.
Filecoin connects those who need space to store data with users who have free space on their hard drives. Users who provide storage space are paid with the FIL token.
DIMO
Based on the idea that your information can be useful for many purposes, DIMO offers the possibility for users to monetize their own vehicle and driving data.
Downloading the DIMO app allows users to selectively share information about their cars (such as battery health, trip information, etc.) and be rewarded with the Ethereum- based DIMO token. Services such as used car marketplaces and ride-sharing apps can purchase access to this data to improve their operation.
Hivemapper
Hivemapper aims to provide decentralized real-world mapping by using dashcams to replicate the services provided by Google Street View.
After purchasing a Hivemapper dashcam and installing it in their car, users drive around (during daylight hours) and generate visual data associated with GPS geolocation that can be uploaded to the network. Users are rewarded with the Solana -based HONEY token . Those who wish to use the map can purchase map credits that correlate to distances/areas that have been recorded by the network of users.
DePIN Essentials
DePIN refers to interconnected real-world devices that provide digital services in a decentralized manner.
Cryptocurrency tokens are used by DePIN development projects to incentivize and reward those who use and/or maintain the hardware that powers the networks.
Helium pioneered DePIN, offering decentralized networks for Internet of Things (IoT) devices and 5G cellular data, but other uses for DePIN include cloud storage, geo-mapping, data sharing, and more.
0 notes
Text
Smart Water Meter Market Growth Forecast: Value & Volume Projections with Demand Insights and Competitive Dynamics
The smart water meter market has gained significant traction in recent years, driven by the increasing need for efficient water management systems, advancements in IoT technology, and a growing emphasis on sustainability. These meters offer real-time data collection and analysis, which allows utility companies and consumers to monitor water usage more effectively, reduce wastage, and optimize distribution systems. As the global demand for water management solutions grows, the smart water meter market is poised for substantial growth.

Market Overview and Growth Drivers
The smart water meter market refers to devices that employ advanced technology to measure water consumption, automate data collection, and communicate usage data to utilities or consumers. These meters are often equipped with sensors, communication modules, and analytical software to transmit data, providing insights into consumption patterns and potential leakages.
Several factors are driving the growth of the smart water meter market:
Increasing Demand for Water Conservation: With rising water scarcity issues, particularly in urban areas, governments and municipalities are pushing for smart solutions to optimize water use. Smart meters help detect leaks, monitor consumption, and promote conservation by encouraging consumers to manage water usage more efficiently.
Technological Advancements in IoT and Connectivity: The rise of the Internet of Things (IoT) and advancements in wireless communication technologies like NB-IoT (Narrowband IoT) and LoRaWAN are enabling real-time data transmission. This allows for continuous monitoring and reporting of water usage, ensuring accurate billing and reducing human errors.
Government Initiatives and Regulatory Policies: Many governments are implementing regulations to promote the adoption of smart meters to improve resource management. For example, initiatives that require utilities to deploy smart metering systems to reduce operational costs and enhance customer engagement are fueling market growth.
Urbanization and Infrastructure Development: As cities continue to grow and demand for water increases, the need for modernized infrastructure becomes more apparent. Smart water meters help municipalities manage the growing demand efficiently, allowing for proactive maintenance and reducing the risk of water shortages.
Market Segmentation and Projections
The smart water meter market is expected to see robust growth in the coming years, driven by rising demand across both residential and commercial segments. The market can be segmented by technology type, end-user, and region.
By Technology: The market can be divided into automated meter reading (AMR) and advanced metering infrastructure (AMI) systems. While AMR systems are widely adopted due to their cost-effectiveness, AMI systems are gaining popularity because of their ability to provide real-time, bidirectional communication, offering more comprehensive data and insights.
By End-User: The residential segment is expected to dominate the market as governments push for smart meters in households to promote water conservation. However, commercial and industrial users are also becoming increasingly important, as large-scale enterprises and industries seek solutions to manage their water usage more efficiently.
By Region: North America and Europe are currently the leading markets, owing to high adoption rates driven by strong regulatory frameworks and government initiatives. However, Asia Pacific is expected to experience the fastest growth, with countries like China and India investing heavily in infrastructure modernization and smart city projects.
Competitive Dynamics
The competitive landscape of the smart water meter market is characterized by the presence of several key players, including Sensus (Xylem), Itron, Badger Meter, and Landis+Gyr. These companies are focusing on innovation, product development, and strategic partnerships to gain a competitive edge. Many of them are also collaborating with utilities and municipalities to pilot smart water metering solutions, which further drives market adoption.
Additionally, smaller players are entering the market, offering specialized solutions tailored to specific regions or applications. This increasing competition is leading to the development of more cost-effective and advanced smart water meters, driving further market growth.
Conclusion
The smart water meter market is on a strong growth trajectory, fueled by technological advancements, growing demand for water conservation, and favorable regulatory policies. With increasing investments in smart infrastructure and urbanization, the market is expected to see robust demand across residential, commercial, and industrial sectors. As the market matures, competition will intensify, leading to innovative solutions that will shape the future of water management worldwide.
Get Free Sample and ToC : https://www.pristinemarketinsights.com/get-free-sample-and-toc?rprtdtid=NDU2&RD=Smart-Water-Meter-Market-Report
#SmartWaterMeterMarketForecasting#SmartWaterMeterMarketDemandInsights#SmartWaterMeterMarketCompetitiveDynamics#SmartWaterMeterMarketProjections#SmartWaterMeterMarketValueAndVolume
0 notes
Text
Integrating AI in IoT Devices: A Comprehensive Guide
The Internet of Things (IoT) has revolutionized the way we interact with technology, bringing smart, connected devices into our homes, workplaces, and cities. However, the true potential of IoT can be unlocked when combined with Artificial Intelligence (AI). Integrating AI into IoT devices can enhance their functionality, enabling them to make intelligent decisions, predict outcomes, and learn from user interactions. This guide will explore the steps and considerations involved in integrating AI into IoT devices.
Understanding the Basics
What is IoT?
IoT refers to a network of physical objects embedded with sensors, software, and other technologies to connect and exchange data with other devices and systems over the internet. These devices range from everyday household items to sophisticated industrial tools.
What is AI?
AI is the simulation of human intelligence in machines designed to perform tasks that typically require human intelligence. This includes learning, reasoning, problem-solving, perception, and language understanding.
Why Integrate AI into IoT?
The integration of AI into IoT devices can lead to the creation of smart, autonomous systems that can:
Analyze data in real-time
Improve efficiency and performance
Predict maintenance needs
Enhance user experiences
Automate decision-making processes
Steps to Integrate AI into IoT Devices
1. Define the Use Case
Before starting the integration process, it is crucial to define the specific use case. Understanding the problem you are trying to solve and the benefits AI integration will bring is essential. Examples of use cases include predictive maintenance in industrial equipment, personalized healthcare monitoring, and intelligent home automation systems.
2. Choose the Right Hardware
Selecting the appropriate hardware is vital for the successful integration of AI in IoT devices. The hardware must support AI processing capabilities and handle the computational requirements of AI algorithms. Key considerations include:
Microcontroller Units (MCUs): Suitable for simple AI tasks with low power consumption.
System on Chips (SoCs): Ideal for more complex AI applications requiring higher computational power.
Edge Devices: These are capable of processing data locally, reducing latency and bandwidth usage.
3. Data Collection and Management
AI models rely heavily on data. Therefore, establishing a robust data collection and management system is critical. The steps involved include:
Sensor Integration: Equip IoT devices with sensors to collect relevant data.
Data Storage: Use cloud or edge storage solutions to manage the vast amounts of data generated.
Data Preprocessing: Clean and preprocess data to ensure it is in a suitable format for training AI models.
4. Develop AI Models
Developing AI models involves choosing the right algorithms and training them using the collected data. Common AI techniques used in IoT applications include:
Machine Learning (ML): For tasks such as predictive maintenance and anomaly detection.
Deep Learning (DL): For more complex applications like image and speech recognition.
Natural Language Processing (NLP): For voice-controlled devices and chatbots.
5. Implement Edge Computing
Edge computing involves processing data locally on the IoT device rather than sending it to a centralized cloud server. This approach reduces latency, conserves bandwidth, and enhances data security. Integrating AI with edge computing ensures that real-time data processing and decision-making occur at the device level.
6. Ensure Connectivity
Reliable and secure connectivity is essential for the seamless operation of AI-enabled IoT devices. Options include:
Wi-Fi: Suitable for home and office environments.
Cellular (4G/5G): Ideal for mobile and wide-area applications.
LoRaWAN: Useful for long-range, low-power applications.
Bluetooth: Suitable for short-range communication.
7. Deploy and Monitor
Once the AI model is trained and integrated into the IoT device, it is time for deployment. Continuous monitoring is crucial to ensure the AI model performs as expected and adapts to new data over time. Implement mechanisms for:
Remote Updates: Update AI models and software remotely to improve performance and add new features.
Performance Monitoring: Track the performance of AI models and IoT devices to identify and address issues promptly.
Challenges and Considerations
Data Privacy and Security
With the increased amount of data collected and processed by AI-enabled IoT devices, ensuring data privacy and security is paramount. Implement robust encryption methods, secure communication protocols, and adhere to data protection regulations.
Scalability
As the number of IoT devices grows, scalability becomes a challenge. Ensure that the AI models and infrastructure can scale to handle the increased data volume and processing requirements.
Interoperability
IoT ecosystems often involve multiple devices and platforms. Ensuring interoperability between different systems is crucial for seamless integration and operation.
Power Consumption
AI algorithms can be power-intensive. Optimize AI models and hardware to minimize power consumption, especially for battery-operated IoT devices.
Conclusion
Integrating AI into IoT devices opens up a world of possibilities, from smarter homes and cities to more efficient industries and personalized healthcare. By following the steps outlined in this guide and addressing the associated challenges, you can successfully develop AI-enabled IoT solutions that drive innovation and deliver significant value.
The future of IoT lies in its ability to learn, adapt, and make intelligent decisions, and AI is the key to unlocking this potential. Embrace the convergence of AI and IoT to stay ahead in the rapidly evolving technological landscape.
#embeddedsoftware#embeddedsystems#embeddedtechnology#linux kernel#android#linuxdebugging#androidbsp#aosp#iot development services#iotsolutions
0 notes
Text
How Businesses in USA Can Thrive in the Digital Age with IoT Solutions
In today's rapidly evolving business landscape, the importance of embracing technological advancements cannot be overstated. One such innovation that has become a business imperative is the Internet of Things (IoT). A recent report predicts a staggering $24 trillion investment in IoT solutions annually by 2027, emphasizing the need for businesses to adapt or risk being left behind. Bridgera, a technology company headquartered in Raleigh, USA, understands the significance of IoT and its transformative impact on businesses.
At the core of IoT lies a complex ecosystem of hardware, software, and connectivity. IoT devices serve as sensory appendages, collecting real-time data from the physical world. These devices, ranging from simple temperature sensors to sophisticated industrial equipment, form the foundation of any successful IoT solution.
Connectivity is the nervous system that links these devices together, facilitating seamless communication. Wi-Fi, cellular networks, Bluetooth, and LoRaWAN are just a few examples of the technologies employed for this purpose. The choice of connectivity depends on factors such as range, power consumption, and data transfer rates.
Central to the IoT ecosystem is the IoT platform. This software infrastructure processes and analyzes data from connected devices, serving as the brain of the system. An effective IoT platform enables data storage, visualization, integration with other systems, and the development of IoT applications that transform data into actionable insights and services.
Integrating IoT solutions into core business operations offers several benefits that can help businesses in US thrive:
Increased productivity: By optimizing processes and automating tasks, businesses can achieve significant productivity gains. IoT-enabled sensors provide real-time data on equipment performance, enabling predictive maintenance and minimizing disruptions.
Cost reduction: IoT solutions drive operational efficiency, leading to cost reductions throughout the value chain. Leveraging data analytics, businesses can identify areas for optimization and eliminate wasteful practices.
Enhanced customer experiences: IoT-powered products and services create personalized and engaging customer experiences. For instance, smart devices can provide valuable insights into customer preferences, enabling tailored offerings.
New revenue streams: IoT opens up opportunities for innovative business models. By developing IoT-based products and services, businesses can tap into emerging markets and capture new revenue streams.
While off-the-shelf IoT solutions offer a starting point, developing custom IoT solutions tailored to specific needs is often necessary for businesses to fully realize their potential. Bridgera understands this and offers comprehensive services to empower businesses in their IoT journey.
Key considerations for developing custom IoT solutions include a clear definition of requirements, careful selection of devices that align with objectives and environmental conditions, integration with a scalable and secure IoT cloud platform, robust data analytics capabilities, and prioritizing data protection and device security. Scalability should also be a key design element to accommodate future growth and expansion.
Implementing IoT solutions presents definite challenges, such as complexity, data management, security risks, and demonstrating return on investment. To overcome these hurdles and capitalize on the potential of IoT, businesses in Raleigh require a strategic approach, technological expertise, and a strong partnership.
Bridgera, headquartered in Raleigh, NC, specializes in delivering cutting-edge IoT solutions using connected intelligence. Their services include IoT strategy development, platform selection and implementation, device configuration, data analytics, security and compliance, and ongoing support and maintenance.
In conclusion, for businesses looking to thrive in the digital age, embracing IoT solutions is no longer a choice but a necessity. By harnessing the power of IoT, businesses can increase productivity, reduce costs, enhance customer experiences, and tap into new revenue streams. For comprehensive IoT implementation, Bridgera, with its expertise and commitment to excellence, is the ideal partner for businesses seeking to unlock the full potential of the IoT revolution
0 notes
Text
An In-Depth Exploration of IoT Connectivity Technologies and Their Uses
Discover the top IoT connectivity technologies and their applications in this detailed guide. Learn how to select the right technology for your needs.
Imagine a world where everyday objects communicate seamlessly to enhance the efficiency and convenience of our lives. The Internet of Things (IoT) has revolutionized how we interact with technology. By 2030, it's projected there will be over 32.1 billion IoT devices globally, nearly doubling from 15.9 billion in 2023. Examples include smart home appliances that adjust the temperature upon entry and wearable fitness trackers that continuously monitor health. These devices connect and exchange data thanks to IoT networking technologies. Let's explore these technologies further.
What Are IoT Connectivity Technologies?
IoT connectivity technologies encompass the various methods and protocols used to connect IoT devices to the internet or each other. These technologies enable communication, data transfer, and control for IoT devices, with over 29 billion connections anticipated by 2027.
Why Is IoT Connectivity Important?
The choice of IoT connectivity technology significantly impacts the performance, cost, and scalability of your IoT solution. High-speed, ultra-low latency connectivity is crucial for some applications, making 5G or 4G cellular IoT connectivity ideal despite higher costs and power usage. Conversely, simpler deployments might benefit from low-speed connections, which are more cost-effective and require less power.
Top 5 IoT Connectivity Technologies and Their Applications
Cellular Networks Cellular networks, essential in daily life for phones and tablets, provide wide coverage, reliability, and enhanced security. They are ideal for mobile IoT systems and serve as alternatives to LPWAN technologies like Sigfox and LoRaWAN.
Use Cases:
Point of Sale and Kiosks: Enables vending machines and kiosks to operate without Wi-Fi, expanding retail opportunities.
Delivery Tracking: Provides real-time shipment tracking across diverse environments, including remote or mobile scenarios.
High Data Rate Cellular (3G/4G/LTE/5G) With high Mbps speeds, these networks suit data-intensive IoT applications, mobile devices, and real-time video streaming. They support "massive IoT" deployments, connecting thousands of devices across large areas.
Use Cases:
Fleet Management: Real-time tracking optimizes operations, enhancing route planning and fuel efficiency.
Health Monitoring: Continuous health data transmission from wearables to healthcare providers ensures prompt intervention.
Ethernet Ethernet offers a reliable, low-latency wired connection, ideal where infrastructure is in place. It provides stable performance unaffected by physical barriers, suitable for stationary IoT devices.
Use Cases:
Security Cameras: Ensures uninterrupted video surveillance in homes or businesses.
Game Systems: Minimizes latency for gamers seeking reliable network performance.
Stationary Medical Devices: Facilitates real-time data transmission from medical equipment to centralized systems.
Wi-Fi Common in homes and offices, Wi-Fi supports secure, high-bandwidth connectivity within a limited range. It's ideal for localized IoT ecosystems where devices are confined to a specific area.
Use Cases:
Smart Gadgets: Integrates smart devices like TVs and appliances into home networks.
Digital Signage: Enables content updates and management in commercial settings.
Bluetooth Bluetooth excels in connecting small, battery-powered devices within short ranges, typically up to 30 feet. It offers low energy consumption, widely used in consumer electronics and small-scale IoT deployments.
Use Cases:
Smartwatches/Fitness Trackers: Syncs health data to smartphones, facilitating mobile monitoring.
Bluetooth Low Energy Sensors: Collects and transmits data from sensors in homes or small businesses.
These technologies cater to diverse IoT needs, each offering unique advantages in range, bandwidth, power efficiency, and scalability. The right choice depends on specific application requirements and environmental considerations.
Essential Requirements for Your IoT Connectivity Solution
Each use case has specific requirements translating into particular technology needs:
Technical Requirements: Consider data speed, latency, range, power consumption, and network coverage. High-speed applications might require advanced cellular technologies, while low-power long-range deployments might favor LPWAN solutions.
Commercial Requirements: Costs include initial setup and ongoing operational expenses. Technologies with lower power needs and simpler infrastructure can reduce costs, ideal for large-scale deployments.
Ecosystem Requirements: Compatibility with existing systems, availability of support and development resources, and integration with future technologies are crucial considerations.
Factors to Consider When Choosing IoT Connectivity Technology
Selecting the most suitable connectivity technology involves a careful assessment of each deployment’s characteristics. This strategic decision affects the immediate performance and cost-efficiency of your service and its long-term scalability and future-proofing.
Conclusion
The field of IoT connectivity technologies holds great promise. The IoT ecosystem evolves daily with advancements in low-power protocols, wireless networks, and emerging technologies. Significant advancements in connectivity technology, such as the implementation of 5G networks, will offer extremely low latency and fast speeds, facilitating seamless communication and new possibilities for real-time applications.
0 notes
Text
Things to Know about LoRaWAN Sensors
The Internet of Things (IoT) is rapidly transforming the world around us, connecting everyday objects to the digital realm and enabling a new wave of data-driven applications. In any case, for these applications to flourish, a reliable and efficient way to collect data from a vast network of devices is crucial. This is where LoRaWAN sensors come in, offering a compelling solution for long-range, low-power communication in IoT deployments.
LoRaWAN sensors are tiny, battery-powered devices equipped with LoRa technology, a low-power, wide-area networking (LPWAN) protocol. Dissimilar to traditional Wi-Fi or Bluetooth connections, LoRaWAN focuses on long-range communication and minimal power consumption. This makes them ideal for situations where sensors should be deployed in remote locations or where frequent battery changes are impractical.
The advantages of LoRaWAN sensors are numerous and contribute significantly to the success of various IoT applications. The main advantage is their extended range. LoRaWAN sensors can transmit data over several kilometers, even in challenging environments with obstacles or deep indoor penetration. This vast range eliminates the requirement for dense network infrastructure, making deployment in remote areas like farms, industrial sites, or sparsely populated cities considerably more feasible.
Another key advantage is the deficient power consumption of LoRaWAN sensors. Slowly transmitting little data packets allows these sensors to operate on a single battery for a really long time. This translates to significant expense savings on maintenance and battery replacements, particularly for large-scale deployments with hundreds or thousands of sensors. Additionally, reduced power consumption contributes to a more sustainable IoT ecosystem by minimizing battery waste.
LoRaWAN likewise boasts scalability, allowing a network to grow seamlessly as new sensors are added. This scalability makes it suitable for applications where the number of connected devices could fluctuate or expand over time. Innovative city initiatives, for example, can leverage LoRaWAN sensors for various purposes, starting with a few dozen sensors for air quality monitoring and gradually scaling up to include hundreds of sensors for traffic the board, waste collection, and noise monitoring.
LoRaWAN sensors are a game-changer for the IoT landscape. Their extended range, low power consumption, scalability, security, and two-way communication capabilities make them ideal for various applications. As the world continues to embrace the power of the IoT, LoRaWAN sensors are poised to play a vital role in connecting the physical world to the digital realm, enabling more innovative, more efficient, and data-driven operations across various industries.
0 notes
Text
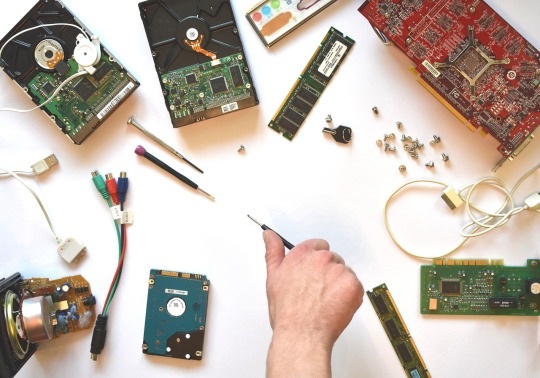
These days, some development boards come with an absolutely astounding variety of hardware. The EESP32, a tiny LoRa board with WiFi, Bluetooth, a transceiver that spans a large portion of the UHF band, and conveniences like OLED displays and an abundance of GPIO, are a perfect example. What about the documentation and firmware, though? Basically, do not say anything at all if you can not say something kind. Better yet, just take a roll. That is not true for every LoRa dev board available, of course, but [Rop] found that to be the case with the Heltec HTIT-WB32LA. This board needed some assistance to get over the line, but it has all the bells and whistles and would be ideal for LoraWAN and Meshtastic applications. Based on his fork of the RadioLib library, which includes a library that significantly lessens wear on the ESP32's flash memory, [Rop] has contributed a fair amount to this end. The library supports all of the hardware on the board, including the pushbutton, display, power management, battery charging, and blinkers, in addition to complete radio support. Many sample applications are included in [Jop], ranging from the minimal requirements to spin up the board to a fully functional spectrum analyzer. It is a wonderful piece of work and a wonderful way to support the LoRa community. And if you want to put one of these modules to work, you’re certainly in the right place. We’ve got everything from LoRaWAN networks to the magic of Meshtastic, so take your pick and get hacking.
1 note
·
View note
Text
Why Choose LoRaWAN Over WiFi, Bluetooth, Zigbee, Cellular, and Others?
Wireless protocols like WiFi, Zigbee, and Bluetooth that operate in the crowded 2.4GHz spectrum often fall short when it comes to supporting wireless sensors for several reasons. Firstly, 2.4GHz protocols have limited range and struggle to penetrate obstacles like walls and floors. You've probably experienced difficulty maintaining a WiFi connection on the second floor when your router is in the basement with obstructions in between. Home automation systems using Zigbee, for instance, may encounter connectivity issues even within the same room.

In contrast, LoRa devices can cover vast distances, even in open environments, and perform well even when there are buildings or equipment in the way.
Secondly, the 2.4GHz spectrum is typically crowded with various devices competing for airtime, leading to reduced link quality. LoRa operates at 915MHz in the US, avoiding interference with local WiFi and most other wireless devices.
Thirdly, security and key management can be problematic with protocols like WiFi. If you change the password on your WiFi router, all WiFi devices need to be updated accordingly. But how do you update the password on small, battery-powered devices that lack a user interface? Common devices like smartphones, TVs, and laptops have displays and allow for easy password changes, but this isn't the case for simple battery-powered sensors.
LoRaWAN, however, handles device provisioning and security differently. Instead of relying on a single password set at the network server, the key originates at the sensor itself and has a unique value that can be provisioned at the network server, often in the cloud. Radio Bridge sensors, for example, come with unique ID/Key pairs, enabling efficient provisioning and security management.
Fourthly, devices like WiFi, Bluetooth, Zigbee, and cellular devices consume relatively high amounts of battery power. They not only use high transmit power but also need to maintain continuous communication with a gateway or base station to stay connected. In contrast, LoRaWAN devices can enter a deep sleep mode and only wake up when necessary to transmit data, resulting in significantly longer battery life, typically ranging from 5 to 10 years in most applications.
0 notes
Text
Exploring The Power Of Edge Computing: Revolutionizing Data Processing In IoT
Welcome to the future of data processing! In a world where our devices are constantly connected and exchanging vast amounts of information, there’s one groundbreaking technology that is revolutionizing the way we handle data: edge computing. Forget about relying on distant cloud servers or slow network connections – edge computing brings the power of processing right to the source, unleashing new possibilities for smart homes, autonomous vehicles, industrial automation, and so much more.
Join us as we dive into this cutting-edge phenomenon and uncover how it’s reshaping the landscape of IoT (Internet of Things) with lightning-fast speed and unparalleled efficiency. Get ready to be amazed by the potential unleashed when data meets its match in edge computing!
Introduction to Edge Computing
As the Internet of Things (IoT) continues to proliferate, so does the need for edge computing. Edge computing is a type of distributed computing that brings computation and data storage closer to the devices and sensors that collect and generate data. By moving data processing and analysis closer to the edge of the network, edge computing can help reduce latency, conserve bandwidth, and improve security.
In many cases, data collected by IoT devices needs to be processed in real-time in order to be useful. For example, if an IoT sensor detects a leak in a pipe, it needs to be able to notify the relevant authorities immediately so they can take action to fix the problem. If the data has to travel all the way back to a central data center for processing, there would be significant delays that could result in costly damage. By processing data at the edge, near the source of collection, real-time responses can be achieved.
Similarly, bandwidth constraints are another common challenge faced by IoT deployments. Since most IoT devices are constrained in terms of power and resources, they often rely on low-bandwidth connections such as cellular or LoRaWAN. Sending large amounts of data back to a centralized location for processing can quickly exhaust these limited resources. By performing data processing at the edge, only the results need to be transmitted back to the central location, conserving bandwidth and reducing costs.
Security is another important consideration for any IoT deployment. The fact that data is being collected from potentially hundreds or thousands of distributed devices makes it a prime target for malicious actors. By performing data processing at the edge, organizations can ensure that sensitive data is never exposed to the public internet.
Overall, It provides many benefits for IoT deployments, including reduced latency, improved security, and conserved bandwidth. As more businesses look to leverage the power of IoT technology, edge computing will continue to gain in importance.
Benefits of Edge Computing
As the world becomes increasingly connected, the volume of data being generated is growing at an exponential rate. It is a new technology that promises to revolutionize the way data is processed in IoT (Internet of Things) applications.
It brings the compute power and storage closer to the data source, which reduces latency and improves performance. It also enables real-time processing of data, which is critical for many IoT applications such as autonomous vehicles, industrial control systems, and smart cities.
There are many other benefits of edge computing including improved security, lower costs, and increased scalability. In this blog post, we will explore the power of edge computing and how it can be used to revolutionize data processing in IoT applications.

What is IoT and Why is it Important?
The Internet of Things, or IoT, is a network of physical objects that are connected to the internet. These objects can include anything from everyday items like watches and TVs to industrial machines and vehicles. By 2020, it is estimated that there will be over 20 billion IoT devices worldwide.
IoT provides a way for these objects to share data and interact with each other. This has a number of benefits, including:
– Improved efficiency: By collecting data from all the devices in an IoT system, it is possible to optimize performance and reduce costs. For example, an energy company might use IoT to monitor electricity usage in real-time and make adjustments to the power grid accordingly.
Increased safety: By monitoring conditions in real-time, IoT can help to prevent accidents. For example, a traffic management system might use sensors to detect an accident and then send information to nearby drivers so they can take alternate routes.
Better decision making: With access to data from multiple sources, decision makers can receive accurate, up-to-date information when they need it. For example, a retailer might use IoT data to track customer behavior patterns and then adjust inventory levels accordingly.
IoT is already having a major impact on businesses and society, and this is only set to increase in the future. Edge computing is one of the key technologies that will enable IoT systems to reach their full potential.
Understanding the Role of Edge Computing in IoT
IoT devices are becoming increasingly sophisticated, and as a result, the data they produce is becoming more complex. In order to make sense of this data, it needs to be processed quickly and efficiently. This is where edge computing comes in.
It is a type of distributed computing that brings data processing closer to the source of the data. By doing this, it reduces latency and improves performance. It also offers a number of other benefits, such as improved security and privacy, as well as reduced costs.
It is playing an important role in IoT, and its importance is only set to increase in the future. With the help of edge computing, IoT devices will be able to process data more effectively and provide insights that can help improve our lives.
Challenges to Overcome with Edge Computing
The challenges to overcome with edge computing are many and varied. Perhaps the most significant challenge is the need for improved data processing speed and efficiency. With the vast majority of data being produced by IoT devices, the volume of data that needs to be processed is increasing exponentially. This requires new approaches to data processing, which can be provided by edge computing.
Another challenge is the need for real-time data processing. Many IoT applications require data to be processed in real time in order to be effective. This can be a challenge for traditional centralized data processing architectures. Edge computing can provide the required real-time data processing capabilities.
Security is another important challenge that needs to be addressed with edge computing. With IoT devices often collecting and transmitting sensitive data, there is a need to ensure that this data is properly secured. Edge computing can help to improve security by providing local data processing and storage capabilities.
Scalability is an important challenge for edge computing. As the number of IoT devices and applications continues to grow, the demand for edge computing resources will also increase. It is important to ensure that edge computing solutions are scalable in order to meet this growing demand.
Examples of Edge Computing in Action
Edge computing is already revolutionizing data processing in IoT, and the potential applications are vast. Here are some examples of edge computing in action:
Smarter traffic lights that can adapt to real-time conditions and optimize traffic flow
Cameras that can immediately detect security threats and send alerts
Intelligent industrial robots that can make decisions without waiting for a connection to the cloud
Connected devices that can diagnose problems and take corrective action without human intervention
Wearables that can provide real-time feedback and coaching to improve user performance
These are just a few examples of how edge computing is making a difference in the world of IoT. As data processing moves closer to the source, we can expect even more amazing applications of this technology in the future.
Conclusion
Edge computing has enabled us to go beyond traditional data processing and reach new levels of efficiency, flexibility, and scalability in IoT systems. By utilizing distributed computing power at the edge of networks, businesses are now able to process data closer to its source. This is a major shift from depending on centralized cloud-based solutions for processing real-time events happening on the ground level. With the continued development of powerful edge technologies, we can only expect these benefits to further increase as more companies move towards an edge infrastructure.
0 notes
Text
How to choose LoRa module product
The LoRa module has become a rising star of the Internet of Things communication technology with its "long distance, low power consumption" advantage. With its obvious advantages: large-capacity, global unified standards, free frequency bands, low cost and flexibility, like WiFi, it has become the primary choice of "private Internet of Things" (NB-IoT, like GPRS, is "public objects," public objects. The solution of the Internet). How to choose the most suitable LoRa module product to become the top -level design of the Internet of Things. For this reason, we discuss together.
1. Construction elements
Demand is the source of the project! In the same way, building an Internet of Things first requires the following needs:
1.1 Distance
Because of the long -distance characteristics, the mainstream of the LoRa Internet of Things is the "star network", which means that the distance refers to the communication distance between the farthest node and the gateway, as shown in the figure above.

Under the same conditions of "transmitting power + communication rate + antenna", the communication distance of the LoRa module is seriously dependent on terrain and environment, such as: high altitude gas balloons reaches 40km; 2 mountain heads or iron tower communication reaches 15km, and communication in open areas has reached reaching the open area communication reaches the open area. 5km ... Because the wireless communication environment is different, it can only be based on the "empty vision"; other environments shall prevail.
3 methods with insufficient communication distance:
① Reducing the communication rate can increase the receiving sensitivity;
② Replace the high-gain antenna and adjust the direction of the antenna;
③ Increase the gateway and effectively cover the signal blind spots.

LinkLabs announced a method of calculating the LoRa network distance. As shown in the figure above, it is very interesting. Drag some variables on the left, and the effective communication distance will be automatically calculated on the right.
The link of this method is: https://www.link-labs.com/symphony
1.2 Scale
The scale is the popular saying of "node number", which is a variable that is easy to count.
1.3 Bandwidth
The bandwidth here, the more popular name is the demand for "network throughput", and its unit is "Bit per second".
Such as: 100 nodes, each node, 37 bytes are reported every 60 seconds, because the Lorawan protocol generally needs to add 13 bytes of "metadata" (frame head and test), then the "bandwidth" required is:
(37 + 13) X 8 bit / 60S x 100 = 667 bps
1.4 Power consumption
If the terminal and sensor (or the driver) are powered by the battery, energy saving will be an important indicator.
Thanks to Lorawanclass A's "Synchronous" feature, the end of the terminal is very good.
Generally, energy consumption is calculated by "mode + current + duration".
Taking the Lorawan node of Rymi as an example, in different working mode, the power consumption is as follows:
Dormation = 1.6ua, listening = 13mA, launch (17dbm) = 88mA.
There is a LORAAWAN terminal and sending a data frame for about 10 minutes, about 1000ms; according to the agreement, after the sending, it can be awakened within 1 second. The listening time is 160ms and the receiving time is 1000ms; other times are in Sleep dormant. In 10 minutes (600 seconds), the energy consumption is as follows:
Send: 1000ms *88ma = 88mA.S
Receive: 1160ms *13ma = 15.08ma.s
Dormation: (600-1-1.16) S* 1.6ua = 0.96ma.s
Average power consumption: (88 +15.08 + 0.96) MA.S / 600S = 0.17ma
The total electrical energy of AA batteries (Nanfu or Shuanglu) is about 2400mAh, and the time for working is: 2400mAh / 0.17ma = 14118h = 1.6 years.
1.5 Topology
When the scale of network nodes increases, this requires more gateways to support it. This will use the "multi -star type" network -level connection. For details, please refer to the "Middle/Large Lorawan" below.
1.6 Cost
Cost is a more complicated topic. In addition to formal costs (procurement equipment, deployment construction, etc.), it also has intangible costs (development debugging, technical support, etc.).
A simple method of calculating costs is to get all the costs of a LoRa IoT (formal cost + intangible cost). Except for the number of nodes, you can get "single -point construction costs".
There are 2 basic principles for LORA IoT costs:
Large quantity: This is the basic principle of the market economy, not explained.
Industry maturity: The industry is high in the early stage, because cost (R & D/manufacturing/marketing/support, etc.) has not been "diluted".
2. Point -to -point (single -cell animal)

The LoRa point -to -point system has a small amount of application scenarios in reality, such as: use the handheld machine to "name" the energy meter meter (electric/water/gas/hot meter), remote control valve, etc. Of course, its limitations are obvious:
1. There is no avoidance mechanism: there is no LBT (Listenbeface Talk) mechanism, if 2 or more nodes are sent at the same time, the radio signal will be damaged and the communication fails;
2. Receive nodes cannot be low power consumption: receiving nodes must wait for the signal of the node at any time to sleep;
3. Unable to automatically organize network: Can't solve conflict and low power consumption, networking has become the air tower.
3.TDMA (Fish Age)
If the needs of networking meet the following conditions, you can use the Lora-TDMA system.
1) The number of nodes is small;
2) There is a regular law of reporting and issuing communication;
3) The requirements for bandwidth are very low.

The advantage of LoRa-TDMA is: low cost implementation of small-scale networking.
At the same time, its disadvantages are also obvious: network capacity is limited, and delay increases linearly with the number of nodes. As shown in the figure below, when n = 10, a node needs to wait for (10 x slot) to be reported before reporting; when n = 100, you need to wait for the time of (100 x slot) before the report is allowed.

4. Small Lorawan (Dinosaur Age)
If the number of nodes is small, but there are requirements for real -time and throughput, it is a suitable solution to choose a small Lorawan.
Its advantages are: 8 channels, allowing 8 nodes to be reported at the same time; standards are unified, and the equipment of each manufacturer can be connected.
If you look closely, you will find that in the Lorawan architecture, there is always the existence of Lorawan Server. It brings complexity, although the cloud server can only reduce complexity.
Small Lorawan: Server Localization

Small Lorawan: Server Cloud

5. Mid -size Lorawan (mammalian)
When you need to improve "real -time" or "network capacity", adding the LoRaWan gateway is a good choice.
As shown in the figure below, adding one or more gateways in a LoRaWan network will not bring any conflict. Because it has only one "brain" -LoRaWan Server, it will execute the following "smart" logic:
Report to reduce redundancy: If a packet is received by multiple gateways, Server will recognize this "repeated" packet based on ID and FCNT, only one of the copies.
Selected gateway: Server always selects the right gateway (often the "best signal strength"), allowing it to launch down the data frame.
Middle -size Lorawan: Server Localization

Middle -size Lorawan: Server Cloud

6. Large Lorawan (Modern Humans)
The original intention of Lorawan-the "telecommunications-level" IoT for regions and countries and the world, which is an ambitious plan.
As shown in the figure below, with the help of 3G/4G technology, many Lorawan gateways are connected to Server; Customer server provides massive storage and intelligent computing; convenient data access and interaction for authorized terminals (PC, smartphones, tablets, etc.).
At present, LoRa and NB-IoT (China), EMTC (US), SIGFOX (France) and other telecommunications-level solutions have strong competition; at the same time, it provides valuable solutions for the construction of "large-scale IoT".
Large Lorawan: Server localized

Large Lorawan: Server Cloud

0 notes
Text
Investigating IoT Network Applications In view of WPAN and LPWAN: A More Critical Glance at XNode Prime

Introduction
The Internet of Things (IoT) has reformed the manner in which we cooperate with technology, empowering consistent network and information trade between gadgets. To accomplish this availability, different wireless conventions have arisen, each taking care of explicit use cases and prerequisites. In this article, we will dive into two unmistakable IoT availability arrangements: Wireless Personal Area Networks (WPAN) and Low-Power Wide-Area Networks (LPWAN). Moreover, we will investigate the interesting capacities and uses of a state-of-the-art IoT gadget, the XNode Prime, that influences both WPAN and LPWAN innovations.
I. Grasping WPAN
WPAN alludes to a short-range wireless correspondence technology that works with the association between gadgets in a restricted geological area. One of the most notable WPAN principles is Bluetooth, which empowers a consistent network between gadgets, for example, cell phones, workstations, and wearables. Bluetooth offers low power utilization, high information move rates, and a basic matching cycle, making it reasonable for a wide scope of uses, including sound streaming, home robotization, and well-being checking.
One more WPAN standard building up forward movement in IoT applications is Zigbee. Zigbee works on low-power, network-organizing standards, allowing gadgets to frame a self-sorting-out network. With its low power utilization and backing for countless gadgets, Zigbee is normally utilized in home mechanization, brilliant lighting, and modern observing frameworks.
II. Revealing LPWAN
LPWAN, then again, takes special care of long-range IoT networks with low power utilization and expanded battery duration. These networks empower gadgets to send information over tremendous distances while consuming negligible energy. LPWAN advancements succeed in situations where gadgets are conveyed in remote or testing conditions and require long-range correspondence abilities.
One of the most widely taken on LPWAN advancements is LoRaWAN (Long Reach Wide Area Organization). LoRaWAN gives magnificent inclusion, allowing gadgets to convey north of a few kilometers. It offers low power utilization, long battery duration, and the capacity to infiltrate through snags. These elements make LoRaWAN ideal for applications, for example, resource following, shrewd horticulture, and ecological observing.
NB-IoT (Narrowband IoT) is another conspicuous LPWAN technology that works on authorized cell networks. NB-IoT brags about general security, profound entrance, and expansive inclusion. Especially reasonable for applications that require powerful availability, like shrewd urban areas, utilities, and modern mechanization.
III. Presenting XNode Prime: The Combination of WPAN and LPWAN
XNode Prime is an imaginative IoT gadget that combines the capacities of WPAN and LPWAN, offering an exhaustive answer for different IoT applications. This gadget goes about as an extension, empowering consistent correspondence between WPAN gadgets (Bluetooth and Zigbee) and LPWAN networks (LoRaWAN and NB-IoT). We should investigate the vital highlights and utilizations of the XNode Prime:
a. Double Availability: XNode Prime backings both WPAN and LPWAN advancements, allowing gadgets utilizing various conventions to easily convey and trade information. This adaptability is especially important in situations where different wireless principles are utilized.
b. Wide Scope of Utilizations: With its capacity to connect different network innovations, XNode Prime opens up a wide scope of IoT applications. It tends to be used in savvy homes, building computerization, resource following, natural checking, medical care, and then some.
c. Expanded Battery Duration: The XNode Prime is intended to advance power utilization, guaranteeing enduring activity without regular battery substitutions. This component is essential for applications where gadgets are sent to remote or unavailable areas.
d. Information Security: XNode Prime focuses on information security, giving encryption and validation instruments to shield delicate data. This guarantees secure correspondence across different associated gadgets.
IV. Genuine Use Cases
To delineate the viable utilizations of the XNode Prime, we should investigate two genuine use cases:
a. Savvy Horticulture: In a brilliant farming situation, the XNode Prime can interface wireless sensors that screen soil dampness, temperature, and stickiness (by means of Zigbee) to a unified stage. This information can be sent over LoRaWAN, empowering ranchers to go with informed choices in regard to the water system, crop wellbeing, and asset of the executives.
b. Modern Mechanization: In a modern setting, the XNode Prime can connect Bluetooth-empowered gadgets, like mechanical frameworks or modern sensors, with a focal control framework. This reconciliation works with continuous observation, information assortment, and controller, upgrading efficiency, productivity, and well-being in the modern climate.
Conclusion
WPAN and LPWAN advances have altered IoT networks, taking special care of various use cases and necessities. The XNode Prime, a state-of-the-art IoT gadget, joins the qualities of WPAN and LPWAN, empowering consistent correspondence and incorporation across different conventions. With its double network, broadened battery duration, and wide scope of uses, the XNode Prime opens up additional opportunities for IoT organizations. As the IoT environment keeps on growing, the union of WPAN and LPWAN advancements will assume a vital part in understanding the maximum capacity of associated gadgets and changing different businesses.
0 notes