#Quantum Computer
Explore tagged Tumblr posts
Text

this thing makes my dick tingle
84 notes
·
View notes
Text
I looove that quantum computers look, you know, LIKE THAT ™

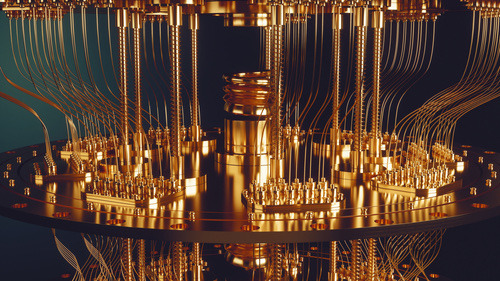

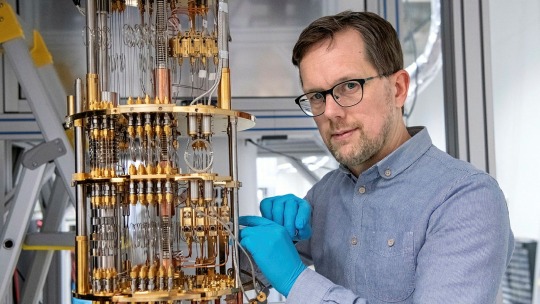
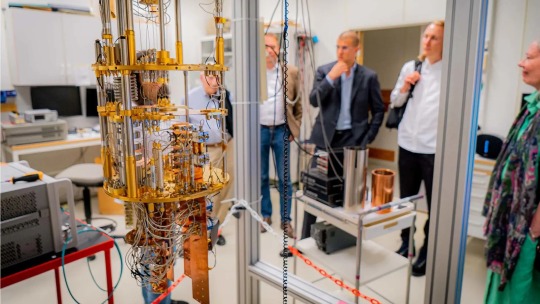




Like a clockwork waiting to chime in a new age (or a bell who may toll for all of us).
Next step is pentagrams and runes instead of circuits.

How many angels can dance on top of a qubit chip? Immeasurable
510 notes
·
View notes
Text
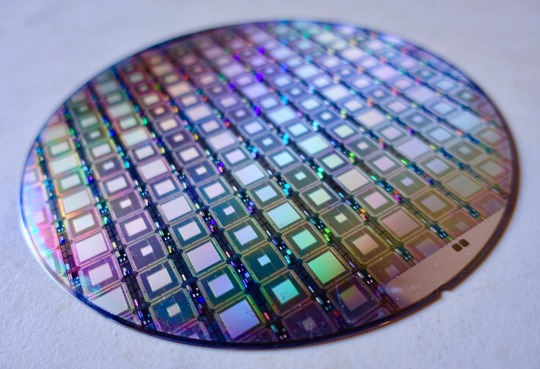
Quantum computing
#wafer#semiconductor#silicon wafer#quantum computing#quantum computer#wikipedia#wikipedia pictures#tech#technology#techcore#technologycore#computing#computer engineering#science
186 notes
·
View notes
Text
Attack for ~Amethyst on Artfight!
#unionised#art#yanska art#digital art#oc#original character#procreate#tech#electronics#quantum computer#digital drawing
15 notes
·
View notes
Text
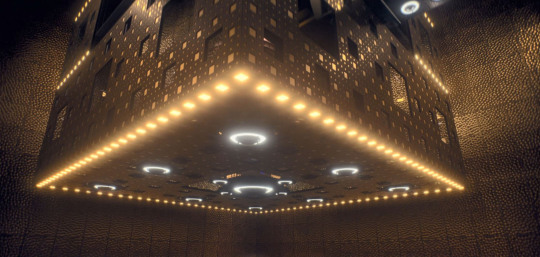
In some respects, one can think of a quantum computer today as being analogous to an analog computer from years ago. The cooling is key to reduce energy and thus vibration in the system. If a quantum computer is run for too long the processor heats up and the noise in the results increases. So sensitive is the computer to heat or vibration that at the $150 million Nanoscience Hub at Sydney University, scientists have to use stairs rather than the lifts because the quantum computer would feel the vibration of the lifts in the building and produce meaningless results. Thus in Devs, the quantum computer main lab space is depicted as a suspended hovering isolated block, inside a bunker style building. This art directed visual feature, like so many in Devs, had one foot in reality and another in fiction.
#devs#devs 2020#alex garland#quantum physics#quantum computing#quantum mechanics#science fiction#Sonoya Mizuno#Nick Offerman#Jin Ha#Zach Grenier#Cailee Spaeny#Stephen McKinley Henderson#Karl Glusman#Alison Pill#software engineering#free will#determinism#quantum computer#dneg#amaya#tech company#faraday cage#many worlds#De Broglie–Bohm theory#science#physics#Socratic method#philosophy#special effects
6 notes
·
View notes
Text

Ink Studio and Benedict Redgrove / Rigetti Computing / Quantum Computer / Photography / 2023
54 notes
·
View notes
Text
The main question is not whether the quantum computer will break Bitcoin encryption and other digital currencies. We already know it will happen. The essential question is: Will digital currency bear the speed, the short time with which the quantum computer will break its encryption?
3 notes
·
View notes
Text
Was Forest’s original plan always to project himself into the machine at the end? It’s always his plan, because this is how he gets to actually be with his daughter again, rather than just watch his daughter. The thing that changes for Forest is that he has adhered to a view of quantum mechanics that does not include many worlds. There’s just one world, which means he can recreate his daughter exactly as she was, and rejoin his life exactly as it was without the car crash happening. What he is forced to accept in the end is that there will be versions of him that can experience that, but also versions that will not experience that. So he has a more poignant end result than the one he was looking for.
So even though he believes in determinism, Forest was going to be able to craft a version of reality that was exactly the same except for the car crash, and go there? That’s exactly right. In effect, what he’d be able to do is rejoin that timeline, but not make the phone call to his wife and not, therefore, be the cause of the car crash. And then he’d be able to experience the unfolding of his life exactly as if the thing never happened. And within that state, it would be a world of equal status to the world that you and I are talking in right now. The problem is that the world is not the simple, deterministic world he wants it to be. So he has to accept a different version.
#devs#devs alex garland#alex garland#simulation#emulation#many worlds#theoretical physics#physics#quantum computer#quantum computing#spoilers#devs spoilers#determinism
2 notes
·
View notes
Text
Quantum computing | Why Need | How does Quantum Computers Work?
What is Quantum computing? Quantum computing is a rapidly growing technology that uses the laws of quantum mechanics to solve complex problems that are not easy for supercomputers. But why do we need Quantum Computers? There are some problems for which even supercomputers are not enough. When engineers faced challenging difficulties in solving some issues, they used supercomputers.…

Classical computers struggle with complex problems like encryption, drug discovery, and AI modeling due to their limited processing power. Quantum computers can solve problems exponentially faster, making them essential for scientific breakthroughs and advanced computing.
How Do Quantum Computers Work?
Unlike classical computers that use bits (0 or 1), quantum computers use qubits, which can exist in multiple states (superposition) and interact through entanglement. This allows them to perform complex calculations simultaneously, making them incredibly powerful for optimization, cryptography, and simulations.
#quantum computing#quantum computer#quantum computing explained#quantum#quantum computing news#quantum computing hype#what is quantum computing#quantum physics#quantum mechanics#quantum computer explained#quatum computing#learn quantum computing#quantum computing course#quantum computing expert#quantum computing qubits#quantum computing design#quantum computing bubble#quantum computing lecture#quantum computing dangers#future of quantum computing
2 notes
·
View notes
Video
youtube
A discussion with Sankar Das Sarma and Chetan Nayak
Mar 14, 2022
Dr. Sankar Das Sarma, a Distinguished University Professor of physics at University of Maryland joins Chetan Nayak, Distinguished Engineer of Quantum at Microsoft to discuss Microsoft’s unique approach to building a fully scalable quantum machine.
#youtube#Chetan Nayak#Sankar Das Sarma#Topology#Quantum Computer#Quantum Computing#Topological Quantum Computer
2 notes
·
View notes
Text
6 notes
·
View notes
Text
I didn't know what I was seeing myself. Lol
That picture of Biden looking at a quantum computer and being unable to grasp the true form of what he's seeing

To be fair that's how I would I react too
44K notes
·
View notes
Text
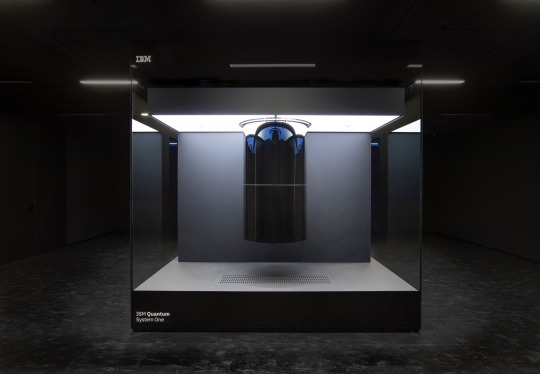
IBM Q System One
#ibm q system one#quantum computing#quantum computer#ibm#wikipedia#wikipedia pictures#tech#technologycore#techcore#technology#International Business Machines Corporation#computer science#computer engineering
38 notes
·
View notes
Text
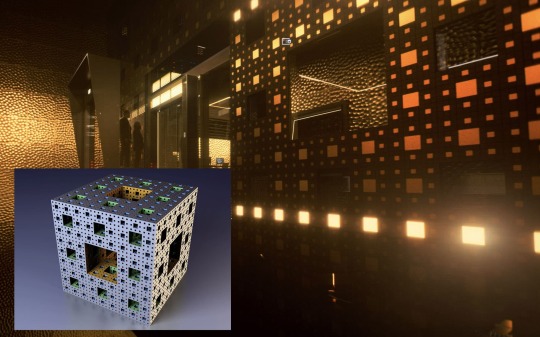
The art direction of the exterior of the inner cube in Devs is based on a Menger Sponge, a complex three-dimensional fractal that illustrates infinite recursive patterns (a mathematical object). “The story of that really goes back to Annihilation (2018) and obviously there’s a lot of fractal imagery in that film, especially Mandelbrot,” Whitehurst comments referring to Director Alex Garland’s previous film.
“That film was still in the back of everybody’s mind… I cannot recall whether the actual screenplay describes the cube as being a Menger Sponge or not? But certainly, I don’t think there was ever any discussion of it being anything else.” The horizontal midsection was built on a set in Manchester and DNEG extended it up and down digitally. On the set, there was also a large trolly that could be pulled back and forth to provide the basis of the capsule that floats across the gap. “Everything above and below that was a very elaborate set extension. Because the lighting is changing the whole time, it was incredibly complicated to do,” explains Whitehurst. The only exception is the major capsule drop in the finale. “That crash is all CG because it had to be,” he explains. “Even for the fully CG shots, wherever we could, we’d get Rob (Hardy : DOP) to operate some sort of camera move to give us something to work with. In those rare cases where we couldn’t do that,” says Whitehurst. “I’ve known Rob long enough that I think I can do a recently good Rob Hardy impersonation of moving a CG camera around!” Hardy and Whitehurst, have both worked many times with Director Garland.
#devs#alex garland#quantum physics#quantum computing#quantum mechanics#devs 2020#science fiction#Sonoya Mizuno#Nick Offerman#Jin Ha#Zach Grenier#Cailee Spaeny#Stephen McKinley Henderson#Karl Glusman#Alison Pill#software engineering#free will#determinism#quantum computer#dneg#amaya#tech company#many worlds#De Broglie–Bohm theory#science#physics#special effects#practical effects#menger sponge#fractal
5 notes
·
View notes
Text
QpiAI Launches 25-Qubit Superconducting Quantum Computer

QpiAI-Indus, a 25-qubit superconducting quantum computer, was launched by Bangalore-based QpiAI, a NQM member. This milestone places India at the forefront of quantum technology development and supports the NQM on World Quantum Day, April 14, 2025.
Full-stack quantum computing system QpiAI-Indus uses quantum hardware, mechanisms, and optimised software. The architecture includes superconducting qubit quantum processors, next-generation Quantum-HPC software platforms, and an AI quantum optimisation tool.
QpiAI's success is due to its selection as one of eight companies for the Department of Science and Technology's National Quantum Mission (NQM). India will invest $740 million in the NQM during fiscal years 2023–2031 to improve its quantum talents in computing, communication, sensing, metrology, and materials. QpiAI launches to assist NQM core infrastructure and enhance quantum technology.
This milestone advances deep-science and deep-tech innovation in biological sciences, medication development, materials sciences, transportation, logistics, sustainability, and climate action by QpiAI.
QPiAI
QPiAI uses modelling, computing, and automation to tackle utility-scale scientific and business problems.
Gen-AI Solutions Enterprise
Use enterprise-grade Gen-AI and AI to transform your business. Integrated systems including AI agents, computer vision, LLMs, and predictive analytics boost automation and decision-making across sectors.
Quantum-powered Options
Solve complex simulation, machine learning, and optimisation problems to advance your industry's use cases, such as manufacturing, healthcare, finance, or science.
Full-Stack Vertical Integration
Easily integrate quantum computing into your AI stack, including hardware and software, to improve modelling, speed up computations, and expand applications utilising next-generation high-performance computing.
Quantum Full-Stack Computing
A hybrid quantum-classical stack with co-located data centres and cloud computing that integrates the greatest AI, software, quantum computer, and classical HPC.
25-Qubit QpiAI Gen-1 Quantum System
Using Superconducting Qubits for enhanced performance, the QpiAI Gen-1 25 Qubit Quantum Computer can scale up to 300 Qubits and has long coherence periods, low error rates, and reliable scaling.
Integrated Quantum Computing System with Single and Multiple QPUs with QPU Virtualisation
Combining AI and quantum technology to help companies solve computational problems.
Smooth integration with high-performance computer technology.
From AI to Quantum
Use AI and quantum computing to solve complex, high-dimensional problems and enhance machine learning, optimisation, and data analysis.
Full-Stack Options
A complete quantum system—software and hardware—designed to integrate smoothly and optimise enterprise applications.
Application Optimisation
Customised quantum solutions for finance, logistics, and medical to meet industrial needs.
QCaaS
With on-demand quantum resources with hybrid quantum-classical capabilities, businesses can develop without investing in infrastructure.
A Quantum Cloud
Secure, scalable cloud-based quantum computing with real-time performance monitoring is ideal for enterprises considering quantum solutions.
Quantum, AI Products
QpiAI-Quantum
The end-to-end Quantum Developer's Platform includes the QpiAI-Gen-1 Quantum Computer with Cloud IDE, Quantum Development SDK, and corporate application-oriented QC procedures.
Manage companies, jobs, and credits.
Enterprise-level security and compliance.
Use Application-Specific Circuit Optimisations.
QpiAI-Pro
QpiAI-Pro is the most collaborative AI model creation, development, and production platform. For cutting-edge AI technologies, run the platform with features adapted to your enterprise data centre.
Collection includes over 200 basic models.
Place models in the cloud, data centre, or edge.
Monitor and observe real-time models.
QpiAI-Opt
High-performance combinatorial optimisation solver for scientific and business applications with millions of constraints and decision variables.
Quantum-Inspired Ising Solver.
Faster than traditional optimisation.
Tenfold scalable.
QpiAI-Matter
AI-accelerated discovery platforms for modelling, simulation, and analysis of new functional materials allow teams build and locate application-specific materials 10 times faster.
1000 times quicker than DFT.
AI-accelerated Discovery Workflows: 10+.
QpiAI-Pharma
Quantum-ready and AI-enabled workflows for drug development computational chemistry on conventional AI HPC and quantum computers.
Quantum-Classical Simulation Combination Models.
Ten-Times Faster Drug Design Pipelines.
1000 times quicker than DFT.
QpiAI-Logistics
AI and quantum-powered supply chain, logistics, and transportation optimisation platform with millions of restrictions and decision variables.
Planning routes cuts mileage by 60%.
Lower fleet size by 47%
Convergence is 72x faster.
#technology#technews#govindhtech#news#technologynews#QpiAI#Quantum Computer#QpiAI-Quantum#QpiAI-Pro#QpiAI-Opt#QpiAI-Matter#QpiAI-Pharma#QpiAI-Logistics
0 notes