#recombinant DNA technology
Explore tagged Tumblr posts
Text
What is Biotech? Unlocking the Power of Biology
âUnlocking the Power of Biology: Biotech Innovations Transforming Our Worldâ Biotechnology: Revolutionizing Industries and Improving Lives Biotech, short for biotechnology, is a rapidly evolving field that combines biology, genetics, and engineering to develop innovative solutions for various industries and aspects of our lives. From healthcare and agriculture to environment and energy, biotechâŚ

View On WordPress
#Innovation#agriculture#biofuels#bioinformatics#biology#biomanufacturing#biomaterials#bioprocessing#biosensors#Biotech#biotechnology#gene editing#gene therapy#genetic engineering#genetics#genomics#healthcare#personalized medicine#recombinant DNA technology#regenerative medicine.#science#sustainability#synthetic biology#technology
0 notes
Text
Recombinant DNA Technology Market: Insights, Growth, and Future Trends (2024-2034)
Recombinant DNA Technology Market Overview As of 2024, the recombinant DNA technology market was valued at approximately USD 781.53 billion and is projected to reach USD 1,286.42 billion by 2034, reflecting a compound annual growth rate (CAGR) of 5.10% during the forecast period from 2025 to 2034. The recombinant DNA (rDNA) technology market is witnessing rapid growth driven by advancements inâŚ
0 notes
Text
https://app.socie.com.br/read-blog/144372_recombinant-dna-technology-market-analysis-size-share-and-forecast-2031.html
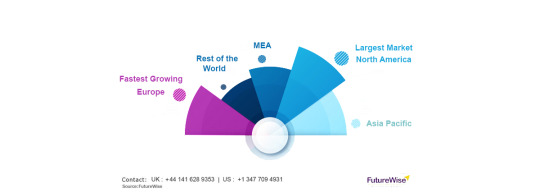
Recombinant DNA Technology Market Analysis, Size, Share, and Forecast 2031
#Recombinant DNA Technology Market#Recombinant DNA Technology Market Scope#Recombinant DNA Technology Market Size
0 notes
Text
As per the report from nova one advisor, the global recombinant DNA technology market size was valued at USD 767.84 billion in 2023 and is projected to reach USD 1,202.46 billion by 2032, growing at a CAGR of 5.11% from 2023 to 2032 according to a new report by Nova One Advisor.
0 notes
Text
As per the report from nova one advisor, the global recombinant DNA technology market size was valued at USD 767.84 billion in 2023 and is projected to reach USD 1,202.46 billion by 2032, growing at a CAGR of 5.11% from 2023 to 2032 according to a new report by Nova One Advisor.
0 notes
Text
The Boston Globe's view of the response at MIT to the decision by the lay members of the Cambridge Experimentation Review Board to allow recombinant DNA experiments to continue in the city.
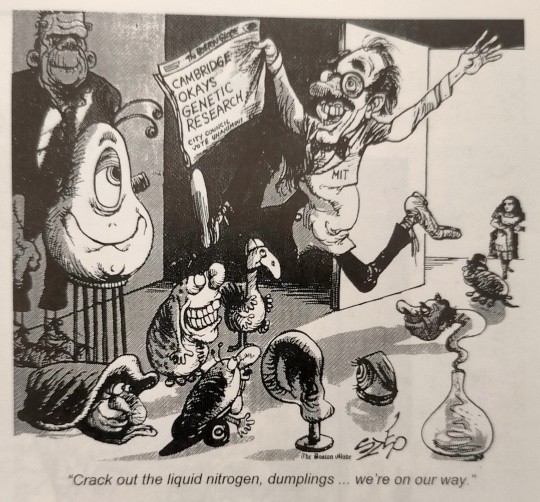
"Frankenstein's Footsteps: Science, Genetics and Popular Culture" - Jon Turney
#book quotes#frankenstein's footsteps#jon turney#nonfiction#boston globe#mit#massachusetts institute of technology#cambridge experimentation review board#recombinant dna#science experiments#liquid nitrogen#genetic research#political cartoon
1 note
¡
View note
Text
Filters in the way of technologically advanced life in the universe and how likely I think they are
1. Abiogenesis (4.4-3-8 billion years ago): Total mystery. The fact that it happened so quickly on Earth (possibly as soon as there was abundant liquid water) is a tiny bit of evidence for it being easy. Amino acids and polycyclic hydrocarbons are very common in space, but nucleotides aren't, and all hypothetic models I've seen require very specific conditions and a precise sequence of steps. (It would be funny if the dozen different mechanisms proposed for abiogenesis were all happening independently somewhere.)
2. Oxygenic photosynthesis (3.5 billion years ago) (to fuel abundant biomass, and provide oxygen or some other oxidizer for fast metabolism): Not so sure. Photosynthesis is just good business sense -- sunlight is right there -- and appeared several times among bacteria. But the specific type of ultra-energetic photosynthesis that cracks water and releases oxygen appeared only once, in Cyanobacteria. That required merging two different photosynthetic apparati in a rather complex way; and all later adoptions of oxygenic photosynthesis involved incorporating Cyanobacteria by endosymbiosis. For all that it's so useful, I don't know if I'd expect to see it on every living planet.
3. Eukaryotic cell (2.4 billion years ago?): Probably the narrowest bottleneck on the list. Segregated mitochondria with their own genes and a nucleus protecting the main genome are extremely useful both for energy production (decentralized control to maximize production without overloading) and for genetic storage (less DNA damage due to reactive metabolic waste). But there's a chicken-and-egg problem in which incorporating mitochondria to make energy requires an adjustable cytoskeleton, but that consumes so much energy it would require mitochondria already in place. Current models have found solutions that involve a very specific series of events. Or maybe not? Metabolic symbiosis, per se, is common, and there may have been other ways to gene-energy segregation. Besides, after the origin of eukaryotes, endosymbiosis occurred at least nine more times, and even some bacteria can incorporate smaller cells.
4. Sexual reproduction (by 1.2 billion years ago): Without meiotic sex (combining mutations from different lineages, decoupling useful traits from harmful ones, translating a gene in multiple way), the evolution of complex beings is going to be painfully slow. Bacteria already swap genes to an extent, and sexual recombination is bundled in with the origin of eukaryotes so I probably shouldn't count it separately (meiosis is just as energy-intensive as any other use of the cytoskeleton). Once you have recombination, life cycles with spores or gametes and sex differentiation probably follow almost inevitably.
5. Multicellularity (800 million years ago?): Quite common, actually. Happens all the time among eukaryotes, and once in a very limited form even among bacteria. Now we'd want complex organized bodies with geometry-defining genes, but even that happened thrice: in plants, fungi, and animals. As far as I know, various groups of yeasts are the only regressions to unicellularity.
6. Brains and sense organs (600 million years ago): Nerve cells arose either once or twice, depending on whether Ctenophora (comb-jellies) and Eumetazoa (all other animals except sponges) form a single clade or not. Some form of cellular sensing and communication is universal in life, though, so a tissue specialized for signal transmission is probably near inevitable once you have multicellular organisms whose lifestyle depends on moving and interacting with the environment. Sense organs that work at a distance are also needed, but image-forming eyes evolved in six phyla, so no danger there (and there's so many other potential forms of communication!). Just to be safe, you'll also want muscles and maybe mineralized skeletons on the list, but I don't think either is particularly problematic. An articulated skeleton is probably better than a rigid shell, but we still have multiple examples of that (polyplacophorans, brittle stars, arthropods, vertebrates).
7. Life on land (400 million years ago): (Adding this because air has a lot more oxygen to fuel brains than water (the most intelligent aquatic beings are air-breathers), and technology in water has the issue of fire.) You're going to need a waterproof integument, some kind of rigid support system, and kidneys to regulate water balance. Plenty of animal lineages moved on land: vertebrates, insects, millipedes, spiders, scorpions, multiple types of crabs, snails, earthworms, etc. Note that most of those are arthropods: this step seems to favor exoskeletons, which help a great deal in retaining water. Of course this depends on plants getting on land first, which on Earth happened only once, and required the invention of spores and cuticles. (Actually there are polar environments where all photosynthesis occurs in water, but they are recently settled and hardly the most productive.)
8. Human-like intelligence (a few million years ago?): There seems to a be a general trend in which the max intelligence attainable by animals on Earth has increased over time. There's quite a lot of animals today that approach or rival apes in intelligence: elephants, toothed cetaceans, various carnivorans, corvids, parrots, octopodes, and there's even intriguing data about jumping spiders. Birds seem to have developed neocortex-like brain structures independently. Of course humans got much farther, but the fact that even other human species are gone suggests that a planet is not big enough for more than one sophont, so the uniqueness of humans might not necessarily imply low probability. (We seem to exist about halfway through the habitability span of Earth land, FWIW.) The evolution of sociality should probably be lumped here: we'll want a species that can teach skills to its offspring and cooperate on tasks. But sociality is also a common and useful adaptation: many species on our list (octopodes are a glaring exception) are intensely social and care for their offspring. I mentioned above that the land-step favors exoskeletal beings, which in turns favors small size; but the size ranges of large land arthropods and very intelligent birds overlap, so that's not disqualifying.
9. Agriculture and urban civilization (11,000 years ago): Agriculture arrived quite late in the history of our species, but when it arrived -- i.e. at the end of the Wurm glaciation -- it arrived independently in four to eight different places around the world, in different biogeographic realms and climates, so I must assume that at least some climate regimes are great for it (glacial cycles are a minority of Earth's history; but did agriculture need to come after glaciations? Maybe a shock of seasonality did the trick). And once you have agriculture, complex urbanized societies follow most of the time, just a few millennia later. Even writing arose at least three times (Near East, China, and Mexico), and then spread quickly.
10. Scientific method and industrialization (300 years ago): We're getting too far from my expertise here, but whatever. The Eurasian Axial Age suggests that all civilizations with a certain degree of wealth, literacy, and interconnection will spawn a variety of philosophies. Philosophical schools that focus on material causes and effects like the Ionians or Charvaka have appeared sometimes, but often didn't win over more supernaturalist schools. Perhaps in pre-industrial times pure materialism isn't as useful! You may need to thread a needle between interconnected enough to exchange and combine ideas, and also decentralized enough that the intellectual elite can't quash heterodoxy. As for industrialization, that too happened only once, though that's another case in which the first achiever would snuff out any other. I hear Song China is a popular contender for alternative Industrial Revolutions (with coal-powered steelworks!); Imperial Rome and the Abbasid Caliphate are less convincing ones. For whatever reason, it didn't take until 18th century Britain.
11. Not dying randomly along the way: Mass extinctions killing off a majority of species happened over and over -- the Permian Great Dying, the Chicxulub impact, the early Oxygen Crisis -- but life has always rebounded fairly quickly and effectively. It's hard enough to sterilize an agar plate, let alone a planet. Disasters on this scale are also unlikely to happen in the lifespan of planet-bound civilizations, unless of course the civilizations are causing them. A civilization might still face catastrophic climate change, mega-pandemics, and nuclear war, not to mention lesser setbacks like culture-wide stagnation or collapse, and I couldn't begin to estimate how common, or ruinous, they would actually be.
****
I have no idea how common the origin of life is, but the vast majority of planets with life will only have bacterial mats and stromatolites. Of the tiny sliver that evolved complex cells, a good chunk will have their equivalents of plants and animals, most of which may have intelligent life at least on primate- or cetacean-level at some later point. At any given time, a tiny fraction of those will have agricultural civilizations, at an even tinier fraction of that will have post-industrial science and technology. Let's say maybe 1 planet with industrial technology out of 100 with agriculture, 100,000 with hominid-level intelligence, 10 million with animal-like organisms, 100 millions with complex cells, and 10 billions with life at all?
178 notes
¡
View notes
Text
Also preserved in our archive
The answer is "by the skin of their teeth," but this article is too polite to say it.
By Joshua Boscaini
COVID-19 is evolving and with it the need for new vaccines to protect people against serious illness and death.
Australia has detected its first cases of the highly transmissible XEC "recombinant" variant â a mix of two previous Omicron variants called KS 1.1 and KP 3.3.
Researchers have been working to ensure immunisations that provide an adequate level of protection against new COVID-19 variants are widely available to the community.
So if there are always new variants, how do scientists keep up with mutations and update the vaccines?
How are mRNA COVID-19 vaccines made? When reports of coronavirus first emerged, researchers quickly obtained a genomic sequence of SARS-CoV-2 â the virus that causes COVID-19.
This helped researchers work out the genetic make-up of the virus and how it causes disease in people, according to the National Human Genome Research Institute (NHGRI).

Once scientists analysed the genetic sequence, they identified the spike protein as the most effective target for the immune system and created a copy or code for it.
Unlike traditional vaccines that contain an inactivated or weakened version of the virus, mRNA COVID-19 vaccines contain a message or code that is delivered to someone's cells.
Doherty Institute professor of virology Damian Purcell said that spike protein code â or RNA message â was "packaged" into lipids to keep it protected for distribution in syringes.
Professor Purcell said once the mRNA vaccine â or message â was injected into a person's muscle, it instructed their cells to reproduce the spike protein.
"These are little bubbles of fat, four different lipids, that together encase the RNA [and] enable it to be protected as it is packaged into syringes and injected into your muscle," Professor Purcell said.
"Those lipids facilitate the uptake and delivery of the essential messenger RNA â the message to be coded within your own cells so your own cells start making the ⌠spike protein."
The process triggers an immune response which creates spike protein antibodies.
The NHGRI said those antibodies remained in the body and recognised the virus if someone became infected, attacking the antigen before it reached healthy cells.
How are vaccines modified to keep up with new strains? Westmead Institute for Medical Research Centre for Virus Research director Tony Cunningham said new strains emerged when the SARS-CoV-2 spike protein changed, making the virus more transmissible.
Professor Cunningham said the mRNA vaccines allowed scientists to change the spike protein code and update the vaccine with the new message.
"If you actually think about RNA like DNA is coloured beads on a string â four coloured beads and they vary along the string â then it's in essence changing that sequence," he said.
"You can just simply change the middle bit of the RNA and that can be done very quickly.
"That spike protein is the one that actually allows the virus to attach to the cell and what we want to do is produce antibodies that stop viruses attaching to the cell."
Professor Purcell agreed, saying one of the advantages of mRNA vaccines was they could be changed and produced usually within a month.
"It's actually one of the really powerful aspects of the mRNA technology, is that many, many steps ⌠can remain the same," he said.
Professor Cunningham said the key to responding quickly to new variants was maintaining good surveillance.
He said it was up to the World Health Organization to recommend what strains should be included in updated vaccines.
Professor Cunningham said the vaccines then needed to be approved by the Therapeutic Goods Administration (TGA) in Australia to make sure they were safe and effective, a process which could take about two months.
Why do vaccines need to be updated? COVID-19 vaccines need to be updated because they cannot protect against newer strains of the virus as effectively, according to Professor Cunningham.
He said that was because the immune system did not have the same antibodies to recognise and fight off the mutated virus.
"Variants can change so they're no longer completely protected against the antibodies that are circulating," Professor Cunningham said.
"That's why we need to keep changing our vaccines, and ⌠particularly in aging people, we need to be immunised every six months to keep the antibodies up."
Professor Purcell said the first Omicron strain was an "escape" variant that required an updated vaccine.
"When the first Omicron came, it had many, many, many changes â more than we'd ever seen before and that was a very significant escape variant," he said.
"People vaccinated with the ancestral strain of vaccine were not protected from transmission with that COVID variant."
He said while people still had some immunity from the original vaccine, it was not enough.
"We do have some underpinning immunity that's capable of still preventing severe disease from those infections but it is still relevant enough to develop a new strain of vaccine," he said.
What vaccines have been approved for use in Australia? Australian Department of Health statistics showed 72.3 million doses of the COVID-19 vaccine had been administered as of October 9.
Pfizer's Omicron XBB. 1.5 and original vaccines were approved for use in children aged five to 11 years old, while Pfizer's original vaccine was also available for children aged six months to four years.
Pfizer's Omicron XBB. 1.5, Original/Omicron BA.4/5 and Moderna's Omicron XBB. 1.5 were available for people aged 12 years and older, according to Healthdirect.
The TGA said it was evaluating Pfizer and Moderna's JN.1 strain vaccine for use in Australia.
#mask up#covid#pandemic#wear a mask#public health#covid 19#wear a respirator#still coviding#coronavirus#sars cov 2#covid isn't over#covid conscious#covid is airborne#covid pandemic#covid19#covidăź19#covid vaccines#covid vaccine#covid vax
38 notes
¡
View notes
Text
Meet our scientists!
ESRA (European Space Research Agency) has officially launched the second phase of its Many Hands project.
With the motto "Manus multae, mens unum" (many hands, one mind), this scientific endeavour seeks to create and maintain small, mobile teams of recombinant scientists in the field on Pandora, in constant communication with their original donor back on Earth.
ESRA thrives to better understand the encironment of Pandora and continue the interrupted research of its many Na'vi cultures and societies.
The RDA recombinant technology allows for scientists to imprint their mind and knowledge onto multiple copies of avatars, who are awakened and brought up to date on recent discoveries while in orbit. They are then sent down to Pandora with their own individual equipment and research goals. Those are solo missions. Each avatar aims to join a local clan or tribe, for the sake of their research and survival. There are no planet-side ESRA facilities, so our researchers depend on RDA air drops for any support, like tech replacement. Communications are maintained via several relays, and discoveries regularly reported to the original human researcher, who then compiles and publishes the finished papers. They work in collaboration with our entire recombinant team to ensure the relevant exchange of knowledge and set new goals.
Eyris Makri is a an exoanthropologist with ESRA. She is part of a 5 bodied recombinant system spliced from TxepĂŹva DNA. Her human self works in collaboration with four recombinants who underwent specialty training during the months of deceleration and approach to Pandora.
Eyris Prime (above), the lead of the mission, specialises on Na'vi communication and published 'Advanced use of volcanic plains stunbat across clans' (2168), 'History and welfare of the working stunbats of the Tau'un clan' (2170) and 'Mythological impact of the domestication and breeding of the viperhounds' (2172).
Every recombinant is deployed with a failsafe (either a neural guillotine or a heart patch), to help ensure there is no repeat of the J. Sully situation. All of our scientists sign up willingly and are sponsored throughout the shipment years. They retain ownership of their DNA and personality inprint after the creation of their recombinants.
See : research on Viperhounds
Research on Stunbats
Both conducted with Serafiina Hukkala (@straydaddy)
#avatar#atwow#avatar 2#jc avatar#avatar fan lore#fanart#concept art#na'vi#na'vi oc#pandora#stunbat#viperhound#txepiva#recombinant#recom#avatar recom#recom oc
37 notes
¡
View notes
Text
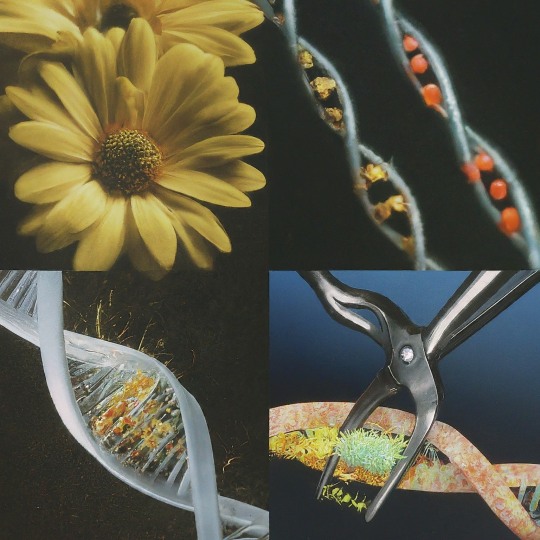
A snip, a splice : Power of rDNA Technology
Deoxyribonucleic acid (DNA), the blueprint of life, holds the secrets to the intricate workings of every living organism. But what if we could manipulate this blueprint, adding, removing, or tweaking its code? This revolutionary concept forms the core of recombinant DNA (rDNA) technology, a powerful tool that has transformed biology and medicine.
The story starts in the early 1970s with two brilliant scientists; Stanley Cohen at Stanford University and Herbert Boyer at the University of California, San Francisco. Cohen, a microbiologist, had been studying plasmids â small circular DNA molecules found in bacteria. Boyer, a biochemist, was an expert on restriction enzymes â molecular scissors that could cut DNA at specific sequences. Their collaboration proved groundbreaking. They envisioned combining these tools to create the first ever recombinant DNA molecule. Cohen provided the plasmids, which would act as vectors to carry foreign DNA into host cells. Boyer, on the other hand, used restriction enzymes to cut both the plasmid and the desired foreign DNA, allowing them to be pieced together. Through meticulous experimentation, they successfully created the first recombinant DNA molecule, forever altering the course of biology.
Cohen and Boyer's work wouldn't have been possible without the earlier discoveries of restriction enzymes. These "molecular scissors" were independently identified by three separate research groups in the 1960s. Werner Arber in Switzerland, along with Hamilton Smith and Daniel Nathans in the US, unraveled the role of restriction enzymes in bacterial defense mechanisms. These enzymes helped bacteria defend against invading viruses by cutting up their foreign DNA. Recognizing the potential of these "genetic scalpels," the groundwork was laid for their application in rDNA technology.
Here's a simplified breakdown of the rDNA process:
Isolation of DNA: The journey starts with isolating DNA from a donor organism.
Cleavage with Restriction Enzymes: Specific enzymes cut the DNA at defined sequences.
Selection of Vector: A carrier molecule (often a plasmid) is chosen to transport the recombinant DNA.
Ligation: The DNA fragments and vector are stitched together using DNA ligase, an enzyme.
Transformation: The recombinant DNA enters a host cell (usually bacteria or yeast).
Selection and Expression: The transformed cells are selected, and the gene of interest is expressed, leading to the desired protein production.
Since its inception, rDNA technology has played a pivotal role in several groundbreaking advancements. Let's take a whirlwind tour through some of the most significant moments in R-DNA history:
1978: Birth of Insulin on the Factory Floor: Scientists achieved a feat of genetic engineering by using R-DNA to produce human insulin in bacteria. This marked a turning point for diabetics, offering a readily available and more consistent source of this life-saving hormone.
1980s: Gene Wars and the Rise of GMOs: The 1980s saw the development of genetically modified organisms (GMOs). Plants were engineered with genes for insect resistance or herbicide tolerance, sparking debates about the safety and ethics of this technology. R-DNA research continues to be at the forefront of discussions regarding genetically modified foods.
1990s: The Human Genome Project Sets Sail: This ambitious international project aimed to sequence the entire human genome. R-DNA techniques played a crucial role in deciphering the 3 billion letters of our genetic code, opening doors for personalized medicine and a deeper understanding of human health and disease.
2000s: Gene Therapy Takes Center Stage: The first successful gene therapy trials for inherited diseases like severe combined immunodeficiency (SCID) took place. R-DNA technology offered a glimmer of hope for treating genetic disorders by introducing healthy genes to replace defective ones.
2010s and Beyond: CRISPR Takes Over: The emergence of CRISPR-Cas9, a revolutionary gene editing tool based on R-DNA principles, has ushered in a new era of genetic manipulation. With unprecedented precision, scientists can now edit genes in various organisms, holding immense potential for gene therapy, crop improvement, and even the eradication of diseases.
But with great power comes great responsibility, and R-DNA raises a host of ethical concerns.Tinkering with the building blocks of life carries the risk of unintended consequences. Engineered genes could escape and disrupt ecosystems, or modified organisms could have unforeseen health effects. The ability to edit human genes opens the door to designer babies, raising questions about social equity and the potential misuse of the technology for eugenics.
Who Controls the Tools? Access to R-DNA technology could be restricted to wealthy nations or corporations, exacerbating existing inequalities. Biosecurity is also a concern, as the technology could be misused for bioterrorism. Creating entirely new organisms forces us to confront what it means to be "natural." Should we modify plants and animals for human benefit, or preserve their original forms? R-DNA technology is a powerful tool, and we must have open discussions about its ethical implications. Scientists, policymakers, and the public all need to be involved in shaping the future of this technology. As we move forward, open dialogue and collaboration between scientists, policymakers, and the public are crucial to ensure the safe and ethical application of this powerful technology.
The journey of rDNA technology is a testament to human ingenuity and its potential to reshape our world. From decoding the secrets of life to creating solutions for healthcare, agriculture, and beyond, rDNA technology continues to evolve, promising a future filled with exciting possibilities.
#science sculpt#life science#science#molecular biology#biology#biotechnology#artists on tumblr#dna#double helix#genetics#recombinant#genetic engineering#insulin#research#education#learning#academics#scientific research#scientific illustration#medical science#scifi#daily dose of science#scientific advancements#scientific tools#medical school
17 notes
¡
View notes
Text
What is Biotech? Unlocking the Power of Biology
âUnlocking the Power of Biology: Biotech Innovations Transforming Our Worldâ Biotechnology: Revolutionizing Industries and Improving Lives Biotech, short for biotechnology, is a rapidly evolving field that combines biology, genetics, and engineering to develop innovative solutions for various industries and aspects of our lives. From healthcare and agriculture to environment and energy, biotechâŚ

View On WordPress
#Innovation#agriculture#biofuels#bioinformatics#biology#biomanufacturing#biomaterials#bioprocessing#biosensors#Biotech#biotechnology#gene editing#gene therapy#genetic engineering#genetics#genomics#healthcare#personalized medicine#recombinant DNA technology#regenerative medicine.#science#sustainability#synthetic biology#technology
0 notes
Text
Day something of productivity
Hello world.
Well that's one way to begin writing something. Isn't it?
I wrote that last night because I was going to make an entry. Well another journal entry.
It's finals.
The second exam of biochemistry is tomorrow.
I need coffee. I hate coffee but I feel like I need it mentally.
What did I get done yesterday?
Nucleotide metabolism
Nutrition
Protein chemistry
Recombinant DNA technology chapter 1
Molecular biology chapter 1
Tumour markers.
What am I going to do today ?
Rest of the syllabus
#mental health#study blog#mental heath awareness#studyblr#academia#undiagnosed adhd#college#school#studying#med studyblr#stem academia#women in stem#stemblr#stem student#med student#med stuff#medstudlife#medblr#actually mentally ill#undiagnosed neurodivergent#probably autism#probably adhd#probably ocd
5 notes
¡
View notes
Text
How I'd Imagine Type 1 Diabetes to be Handled in Teyvat
if anyone has any questions, comments, other ideas, etc, that they'd like to share, by all means go ahead!
possible cws/tws: mentions/non-gorey imagery of needles & cannulas, brief mentions of blood
insulin is made through [bio]alchemy ; I'm not specifically sure which branch would be most likely to take the genetic engineering approach (inspired by how it's made here, typically through genetic engineering or something called Recombinant DNA)
since Fontaine seems to be invention-creation central & the nation that comes out with new creations like the Kamera, they would be responsible for creation of the tech side of having t1d, such as insulin pumps
prior to them creating insulin pumps, insulin was only administered through manual injections (needle tw: 1 / 2)
Fontaine has created the Teyvat equivalents of the following diabetic supplies:
insulin pumps with tubing (1Â /Â 2Â /Â diagram)
lancet devices (which are used to prick yourself and draw blood in order to test your blood sugar) (1 / 2)
meters + testings strips to actually test your blood sugar (meter: 1 / 2 ; strips ; 1)
I doubt they'd be able to create all of the tech we use today, since they don't seem to have the wireless technology & bluetooth to make tubless pumps (1Â ; the only tubeless one that exists at the moment is the Omnipod) or continuous glucose monitors (CGMS) (1 / 2- the sensor is on the left)
however, I'd like to think that if/when they do figure out the technology to actually make those, they'd be made around the same time
Misc. HCs
Lively Orlean is type 1 diabetic (to me) (she mentions her blood sugar being low and while non-diabetics can get hypoglycemic episodes/symptoms [which are caused by a low blood sugar],, come on /lh) (I'd say it's canon but I fear getting yelled at even if I'm right /lh)
Baizhu, being a pharmacist who most definitely sells insulin, is very knowledgeable on diabetes- 1, 2, and the sub-categories-that-idk-much-abt-and-therefore-won't-list
going off vibes alone I'd like to think Verr Goldett also is very familiar with it
maybe either a family member/friend of hers has it, or she herself has it
I think Barbara knows the basics at best but is learning quite a bit about it, primarily how to help treat hypoglycemic / hyperglycemic episodes, Ketoacidosis, and how to administer glucagon
#genshin impact#genshin#genshin headcanons#genshin impact headcanons#disability hcs#genshin hcs#genshin impact hcs#type 1 diabetes#t1d#needles cw#needles tw#blood cw#disability#disability pride#disability pride month#đ | my writing#đ | og posts
23 notes
¡
View notes
Text
Recombinant DNA Technology Market Analysis, Size, Share, and Forecast 2031
#Recombinant DNA Technology Market#Recombinant DNA Technology Market Scope#Recombinant DNA Technology Market Size
0 notes
Text
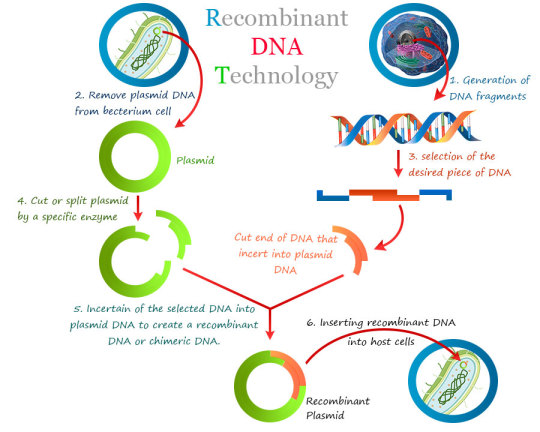
What is Recombinant DNA Technology?
Recombinant DNA technology (rDNA technology) is a process that is used for producing artificial DNA through the combination of different genetic materials (DNA) from different sources.
5 notes
¡
View notes